TITANS OF NUCLEAR
A podcast featuring interviews with experts across technology, industry, economics, policy and more.
Latest Episode

1) The beginning of Brian’s career and his time in the United States Navy as a diver, as well as what drew him to engineering and nuclear
2) Brian’s initial journey to Oregon State and all of the research projects he’s had a hand in since then
3) Fostering a passion for nuclear in the next generation of nuclear engineers and why the researchers as just as important as the research itself
4) What challenges and successes the nuclear industry will face in the coming years and how to form your individual opinion on nuclear energy
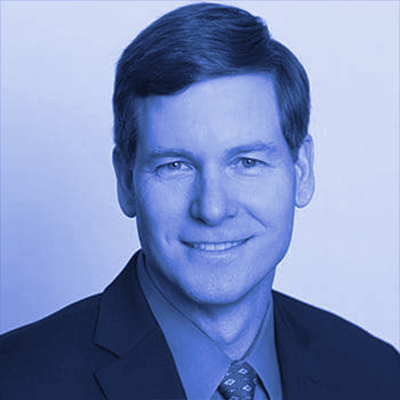
1) John's career at the DOE, the National Labs, as an ANS congressional fellow, and in the private sector
2) NEI's national nuclear energy strategy: Preserve, Sustain, Innovate, and Thrive
3) Pros and Cons of various mechanisms the government can use to support the industry
4) How SMRs alleviate some of the market problems nuclear faces today
"1:53 - Early Nuclear Experience
Bret Kugelmass: Tell us how you got into the nuclear space.
John Kotek: John Kotek grew up outside of Boston and was surrounded by controversies about the startup of the Seabrook plant and the PIlgrim plant, not long after Three Mile Island. Kotek had a physics teacher in high school who talked about nuclear and Kotek’s counselor encouraged him to pursue nuclear engineering. Kotek started out at the Department of Energy (DOE), right before President Clinton cut nuclear energy R&D spending. At the end of the Clinton administration, there was a recognition within the President’s Committee of Advisors on Science and Technology (PCAST) that nuclear belonged in the federal R&D portfolio. By this time, Kotek was working at Argonne National Laboratory and he worked with some colleagues to come up with the idea for a Generation IV nuclear technology roadmap. Kotek spent some time on Capitol Hill as the American Association for the Advancement of Science (AAAS) Congressional fellow through the American Nuclear Society (ANS). He later returned to the DOE as the deputy manager of the field office during the merger of Argonne National Laboratory West and Idaho National Engineering and Environmental Laboratory (INEEL) into the Idaho National Laboratory (INL). After three years, John Kotek transitioned into the private sector but kept getting drawn back to nuclear, eventually getting involved in the Blue Ribbon Commission on America’s Nuclear Future (BRC). One thing the bipartisan BRC worked on was alternatives to Yucca Mountain for nuclear waste management. The BRC recommended building storage capacity into the system to allow the government an opportunity to make good on its commitments to plants and communities to move waste sooner. In late 2014, Kotek got the call to return to the DOE and had the privilege of working with Secretary Moniz.
9:03 - Energy Policy and New Nuclear Technology
Bret Kugelmass: While working with Secretary Moniz, what were some things you learned and what were some challenges you took on?
John Kotek: John Kotek was heartened to see the amount of interest that had grown around advanced nuclear technology. The companies trying to innovate in the space, entrepreneurs, venture capitalists, and NGO’s came together to create an ecosystem around advanced nuclear. Looking back at the history of nuclear technology development in the U.S., there are examples of everything being developed today that was tested years ago. By taking a different approach, the need for backup systems or required staff may be eliminated to come up with a more economic system overall. In the early days of nuclear, facilities were built in a lot less time than it takes to build them today. These new technologies with different fundamental underlying physics and thermomechanical characteristics may provide an opportunity to get to a system that is faster to deploy and less expensive to operate. The faster you can get your asset from construction into revenue production, the better it will be for the bottom line.
12:52 - Nuclear Energy Institute Initiatives
Bret Kugelmass: What does the Nuclear Energy Institute do?
John Kotek: The Nuclear Energy Institute (NEI) represents the nuclear industry, the vast majority of power producers and companies that are suppliers of power plant components. NEI is in the business of trying to get to a greater recognition of the value nuclear brings to the electricity markets and to society as a whole. There is no other technology that offers the combination of resilience, cleanliness, fuel assurance, and price stability that comes with a nuclear plant.NEI’s National Nuclear Energy Strategy has four components: preserve, sustain, innovate, and thrive. The Nuclear Narrative explains the benefits of nuclear to try to bring a broader awareness of the technology. Nuclear power plants are in economic stress in competitive electricity markets, driven by low natural gas prices and renewable portfolio standards. Sometimes it is still profitable for renewables to sell their electricity at a negative price because they make more money from the tax credit. This has been an effective incentive in promoting renewable energy growth, but the unintended consequences of that haven’t been reflected in the way markets price electricity. NEI advocates for a market that values everything that’s being delivered into that market and many attributes of nuclear are not currently reflected in the way nuclear is compensated. For developed in advanced nuclear, technology is important, but the policy framework must be right in valuing low carbon energy. NEI also focuses on delivering the nuclear promise, which is looking for ways to increase the efficiency on the operation side and share lessons learned and best practices across the industry. Part 3, innovate, focuses on advanced reactors and how new technologies can get through the development and licensing process and into commercial use. The fourth parts of NEI’s strategy, thrive, is centered around getting back to the place where the U.S. is competing and winning in world markets against state-owned enterprises such as Russia and China. When you build a nuclear power plant in another country, you start what could be a century long relationship with the country and is an opportunity to spread U.S. safety practices and proliferation standards. Russia and China are viewing the nuclear industries as very strategic. The U.S. needs to ensure their companies compete in effect against countries.
25:50 - International Nuclear Influence
Bret Kugelmass: How can we enable our nuclear companies to compete effectively internationally?
John Kotek: In the history of light water reactor design and development, there has been a history of cost sharing. There are opportunities for the government to continue on the type of cooperation they’ve had with NuScale on the development of the small modular reactor (SMR). Private companies are willing to invest multiples of what the government will invest because they see market opportunities. Power purchase agreements to offtake electricity from a nuclear power plant, particularly a first of a kind or early version of technology, would help accelerate commercialization. There are opportunities to use part of an SMR facility as an experimental facility by using part of an SMR that is built near a National Laboratory as a vehicle to test other energy production from advanced nuclear. Some advanced designs can go beyond electricity production.
30:15 - Small Modular Reactors’ Market Impact
Bret Kugelmass: How does the small modular reactor alleviate some of the electricity and market problems?
John Kotek: A small modular reactor (SMR) design is intended for load following, to meet energy supply and demand. Renewables are looking to energy storage, but since it is not economically viable right now, renewable energy is backed by natural gas. If you don’t need all the electricity an SMR can provide, production can be backed off or part of the output could be used to run an electrolyzer and produce hydrogen or divert some heat for industrial supply. Single digit megawatt SMR’s are very simplified systems that could be transportable and remotely operable. Developing the advanced technology is an essential part in creating a brighter future which takes full advantage of nuclear, but there needs to be broader public acceptance recognition of the value that nuclear delivers and must be conveyed to policymakers and the media. New York, Illinois, and Connecticut have passed state level legislation to preserve their nuclear plants. The closer you get to the nuclear facility, the more people support it and understand it.
36:30 - Innovation in Nuclear Power Plants
Bret Kugelmass: Tell us about some of the people at the Nuclear Energy Institute and efforts they are working on.
John Kotek: The Nuclear Energy Institute’s (NEI) CEO, Maria Korsnick, was responsible for a fleet of nuclear power plants and has lived in communities in which the nuclear power plant was the center of activity in the region. She knows the care and attention that goes into operating these facilities and translates that pride and passion into her advocacy for nuclear energy at NEI. Dan Lipman, VP, is in charge of international trade and suppliers who worked for Westinghouse on the AP-1000’s. When John Kotek got into nuclear in the late 1980’s, nuclear plants were run at 60% capacity factor, the percentage of the hours a year the plant is running. Due to improvements in the way plants have been run and changes to increase power output, there is 40% more energy produced today with fewer reactors running. Accident tolerant fuels (ATF), also known as advanced technology fuels, are an opportunity to develop a new fuel type that can provide better performance and better safety margins. Because of the innovation in fuel development and regulatory steps, experimental fuel rods are going into reactors now. The first application has gone in to take a plant from a 60 year license to an 80 year license and more than 85% of the plants have received approval to operate out to 60 years. The NuScale design started out as the Multi-Application Small Light Water Reactor (MASLWR) program. After the nuclear energy R&D budget when to zero in the late 1980’s, the Nuclear Energy Research Initiative (NERI) was brought back to provide small grants to university and laboratory based researchers. One of the applications was Dr. Jose Reyes at Oregon State University who worked with the Idaho National Laboratory and others on the small light water reactor design. He persevered and 15 years later, their design is in with the Nuclear Regulatory Commission (NRC) and the NRC is validated passive safety features in the design. A new design can be brought from the drawing board into the commercial market. The NRC is looking to make the process more efficient to get to the levels of assurance of public protection they need to demonstrate.
"

1) Jeff's earlier career as an NRC vendor inspector
2) The relationship between NRC and Industry including the balance of health and safety against cost and schedule
3) An alternative safety approach to redundancy and defense in depth
4) The X-energy team composition and its founder Kam Ghaffarian
2:01 - Transition from Oil & Gas to Nuclear
Bret Kugelmass: How did you get involved in the nuclear field?
Jeff Harper: Jeff Harper started as an oil and gas engineer with British Petroleum in Cleveland, Ohio. Harper attended a job fair for the Nuclear Regulatory Commission (NRC) and took a job in the vendor inspection branch as an inspector. This group went out to look at companies like Westinghouse and Babcock & Wilcox to ensure that they complied with their quality programs and supplied the nuclear plants with quality components and services. The NRC has resident inspectors that have offices at the nuclear facilities and are into the day-to-day operations of the nuclear plants. Other inspectors worked out of the headquarters to look at the programmatic side and complete site inspections throughout the supply chain. The U.S. nuclear industry has a superb record for safety and the NRC has been considered all over the world as the gold standard for regulating nuclear activities. The NRC has been classically focused on public safety and health, without much concern to cost and schedule. The agency represents the public’s interest and is both technically and politically oriented. There is a new wave of commercial deployment innovation within nuclear, however, the uncertainty of whether you can or when you will get a license impacts the financing.
8:28 - Global Pebble Bed Technology Development
Bret Kugelmass: How did you move from the Nuclear Regulatory Commission to the private sector?
Jeff Harper: Jeff Harper joined Westinghouse to work with their AP-1000 as a licensing manager in South Africa. Westinghouse was bidding for 9600 MW of Nuclear One against Areva, but the South African government eventually cancelled the procurement due to lack of funding. Harper got deployed into the strategy group inside Westinghouse where he was put in charge of the advanced reactor program. At this same time, Westinghouse was working with the South Africans on the pebble bed reactor. South Africa took the German design and improved upon it in partnership with Westinghouse. This group went forward to develop the pebble bed modular reactor from the improvements the South Africans made to the German technology. The Department of Energy (DOE) has a cooperative agreement program, a public private partnership to help develop the design; X-energy has a similar cooperative agreement now. Because the South African government abandoned the project, the project caved and Harper stayed in South Africa for five years working in the manufacturing business in the oil & gas industry. For Westinghouse, the AP-1000 is bread and butter and they have been on the forefront of engineering and innovation. The pebble bed modular reactor (PBMR) was South Africa’s answer to looking into the future in terms of innovation.
14:49 - Pebble Bed Reactors
Bret Kugelmass: Is the funding for large nuclear plants a problem with historical plant designs?
Jeff Harper: The traditional thinking in nuclear is economy of scale, so the bigger you build, the cheaper it is from a MW/hr standpoint. On the small modular reactor (SMR) side, the industry is looking at economies of volume. The more SMR’s that can be deployed, the cost will come down in terms of component and pressure vessel cost. There is also less concrete and steel and less construction time, which reduces the overall cost of deployment and the operation costs of the facility. Jeff Harper and X-energy are trying to eliminate pumps and valves that aren’t necessarily needed because their design is meltdown-proof. The reactor consists of 220,000 pebbles the size of a billiard ball. Each one goes through the reactor six times in order to get the maximum amount of heat and efficiency. The pebbles have triso fuel which is surrounded by several layers of carbon graphite and silicon carbide; the silicon carbide acts as a pressure vessel within each pebble, so the reactor doesn’t need a containment building. The fission takes place at the triso particle; there are about 18,000 particles within each pebble. The fuel temperatures are never beyond 1100 degrees centigrade. If there was a loss of power and the coolant was shut off, the pebbles would just cool off through natural forces, conduction and convection.
24:12 - Challenges of Licensing Pebble Bed Reactors
Bret Kugelmass: What are the challenges to getting regulatory approval for commercialization of pebble bed reactors?
Jeff Harper: Pebble bed reactors have never been licensed in the U.S., but instead has been light water centric. Because it is meltdown-proof, there are no secondary safety systems, which reduces the complexity of the design. With these changes, the technology must be proved to the Nuclear Regulatory Commission (NRC), but Germany did have pebble bed reactors in the 1960’s. There are some people in the NRC that were trained under some of the original German technology, such as X-energy’s Chief Nuclear Officer, who spent formative years working on the THTR and the AVR German reactors. X-energy has tried to take the best people in the world that were available from the South Africa experience who looked at taking the German technology forward. Dr. Kam Ghaffarian came to the U.S. from Iran, starting with SGT, and engineering services company that became the second largest NASA contractor. Kam has a keen approach for innovation and as he became successful with SGT, began looking at how he can give back to the U.S., leading him to the nuclear side. Advanced reactors have applications that are different than traditional nuclear reactors, such as process heat for water purification and desalination. During Jeff Harper’s time in South African in manufacturing, Harper first learned about Day Zero in Cape Town, which was the day Cape Town would run out of water, currently projected for July 2018 due to a doubling in population and extreme drought.
33:32 - Nuclear Energy’s Impact on Water Purification
Bret Kugelmass: How do you manage the high energy consumption of water purification?
Jeff Harper: X-energy’s reactor design has the ability of co-generation, producing electricity and process heat, due to the operating temperatures. The reactor can produce electricity and provide waste heat from the process to drive the water desalination. The reactor could also be set up to use the steam directly for water processing. Traditional reactors are very large, but X-energy’s reactor only takes up 13 acres of land, allowing them to deploy the technology in areas where it would be very advantageous to do water desal and produce electricity. The plant is physically smaller, approximately 200 MW compared to traditional 1,000 MW plants. These 200 MW plants can also be teamed together in a module to increase capability. Due to inherent safety features, the plant does not need a huge exclusion zone and the emergency planning zone is at the fence of the facility since it doesn’t have source term release. Source term release is the probability of the release of radioactive material.
38:04 - X-energy’s Collaboration with the DOE
Bret Kugelmass: How did X-energy win a $40 million grant?
Jeff Harper: About two years ago, X-energy was successful at a Financial Opportunity Announcement (FOA). This private-public partnership was for a total of $53 million total, with the Department of Energy (DOE) providing $40 million and X-energy providing $13 million. Under this cooperative agreement, the mission was to deploy manufacturing capabilities in the United States for this pebble. X-energy has the reactor design activities, but is also producing the triso fuel for the industry, which will come in different fuel forms. If X-energy didn’t pursue this, China would be the only other source of triso fuel. The fuel business is a near-term company that can be launched within 3-5 years, as opposed to the reactor side, which will take more than five years to deploy. X-energy looks at deployment in three different baskets: technology, licensing, and economics. All three things have to work together to move the company forward. Companies must continue to work with the U.S. government in early days of development and bring in strategic partners that also share the vision and will help finance the project. It is also very important to have a customer in order to get investors. On the licensing side, X-energy must look towards the industry to help with the Nuclear Regulatory Commission (NRC) in regulatory reform. X-energy is preparing themselves to produce a high quality application. It is important to engage with the NRC as early and often as possible. X-energy’s goal is bringing a product to market that is as simple as possible and has a supply chain to market by 2030. Nuclear is complicated, but it’s not all engineering. Nuclear companies need commercial people, financial people, and government relations people. X-energy is confident that their product will make a difference in the world.

1) Jessica's start in Astrophysics, transition to Energy, and how she joined Breakthrough
2) Deployment timelines and how to make nuclear innovative
3) The importance of fuel reliability, export control, and security considerations
4) Canada's efforts on licensing advanced reactor technologies
2:23 - Path from Astrophysics to Nuclear Energy
Bret Kugelmass: How did you transition from astrophysics to nuclear energy?
Jessica Lovering: Jessica Lovering completed her Bachelor’s degree in astrophysics at UC-Berkeley and started her PhD in astrophysics, but has always been passionate about the environment. After living and working in Fairbanks, Alaska after college, Lovering saw the impacts of climate change and became more focused on the big threat of environmental issues. She loved the challenge and problem solving aspect of physics, leading her to switch into an environmental policy graduate program. Energy seemed like the key, but nobody seemed to be talking about nuclear energy and its unique challenges, even though it is the largest source of clean energy. Decarbonization is always hard, but it becomes a lot easier when nuclear is on the table. One of Lovering’s professors was Roger Pielke, Jr., a voice and thought leader in climate known for calling others out for unrealistic assumptions. Through this connection, Lovering ended up doing the Breakthrough Generation fellowship in 2011.
5:51 - The Breakthrough Institute
Bret Kugelmass: What’s the history of the Breakthrough Institute and how did it get formed?
Jessica Lovering: The Breakthrough Institute was founded by Ted Nordhaus and Michael Shellenberger in 2007 as a new way of thinking about environmental problems from a pragmatic approach. There originally was a focus on making clean energy cheap, particularly how the government could invest in clean energy, as described in one of their first papers, “Fast, Clean, & Cheap”. The common thread in Breakthrough’s work is making the best practices for the environment cheaper and easier for people to implement. Breakthrough has always focused on energy and development, but started a conservation program in 2012 and has now opened up into food and farming. Energy, especially nuclear, has always been one of their big focuses. When Jessica Lovering came in to Breakthrough as an energy analyst, she was very interested in nuclear. Fukushima brought it to a head, because it shows how important it is when a country is so dependent on a single energy source. Lovering started doing a deep dive into nuclear and tried to figure out the policies, challenges, opportunities, and feasibility of expanding nuclear in the U.S. and globally. The biggest challenge for nuclear is cost and utilities don’t want to build something that is expensive. However, once it is built, energy is really cheap and it is a great resource. Breakthrough published a report in 2013, “How to Make Nuclear Cheap”, which looked at advanced reactor technologies and explored whether they made nuclear cheaper or whether people were drawn to the technologies for other reasons. Certain attributes of advanced nuclear could make it cheaper, such as whether it can be built modularly, in a factory setting, or built smaller. The AP-1000’s were built with certain modular construction techniques. There is a gradient in how much modularity is used to build nuclear construction. Combined cycle gas turbines, solar panels, and wind turbines are all built in a factory and assembled on-site. Jessica Lovering and the Breakthrough Institute thought about how to turn nuclear from a big megaproject to a product that is delivered to a site.
11:50 - Nuclear Readiness and Innovation
Bret Kugelmass: What does readiness in nuclear mean?
Jessica Lovering: Many advanced nuclear designs were invented at U.S. National Labs in the 1960’s and have been around awhile, but have not been built. For some designs, the right materials or computational power did not exist. Back during the time of design, if you wanted to know what happened in an accident or under different conditions, you had to build an experiment and a model. Today, there is a lot more that can be done with computational modeling now, making it easier to ask “what if” questions and do iterative innovation. In Breakthrough’s report “How to Make Nuclear Cheap”, Jessica Lovering looked at different levels of investment that was required for different technologies to get commercial availability. The industry needs technologies that will be ready soon. In another report, “How to Make Nuclear Innovative”, Breakthrough looked at how other industries innovate, and the “fail fast and fail often” approach turns out to be very different from how nuclear has historically approached innovation. Smaller nuclear plants can test components more rapidly and simpler designs may rely more on safety from physics than from active components.
16:22 - International Construction Costs of Nuclear
Bret Kugelmass: How have construction costs varied in different countries?
Jessica Lovering: There is not an intrinsic trend for nuclear, as there are different trends for countries and time periods. The U.S. is the worst example of cost going very high in a very short period of time, but France was able to keep their cost stable and grew at the same rate as general construction index. South Korea saw their costs higher, but came down as they built more plants. If you are building each plant as a one-off with a different design, different architect-engineering firm, and a different utility that will own and operate it, they are all built as large-scale infrastructure projects. Other countries build the same design is by the same crews and utilities, such as South Korea and Canada. The U.S. usually has 1-2 plants at a site, while South Korea and China have 4-8 reactors which allows economies of scale. The U.S. needs designs that can be built faster and added incrementally, such as small modular reactors (SMR’s). Demand for electricity is flat in the U.S., but there is huge growth and demand for electricity and energy in industrializing countries. A huge benefit of nuclear is fuel reliability. In developing countries, it is really hard to get your coal, natural gas, or diesel delivered consistently year round. Once you have your nuclear fuel, it is two years before you need more and it is a very small quantity.
21:12 - Challenges of Exporting Nuclear Technology
Bret Kugelmass: What are some other challenges that companies have to consider when exporting nuclear technology?
Jessica Lovering: The U.S. does not want nuclear materials and technologies to get into the wrong hands, so there is very good oversight by the International Atomic Energy Agency (IAEA) and most countries are party to the nonproliferation treaty. If countries want to work with a U.S. company in importing nuclear technology or starting a commercial nuclear power program, they go through a process with the IAEA and with the U.S. to sign a 123 agreement. A 123 agreement shows that the U.S. Government has determined that the country is not a security risk and is not going to use the material for weapons or military program. The U.S. is still a major player in equipment and services around the world and it is still important for relationships with different countries. Enrichment is the big worry from a security standpoint and is more a concern about non-state actors. If a government wants to take over a facility and make weapons, it would be very obvious and bold. For non-state actors, such as a terrorist organization, it would be more about sabotage or stealing material to make dirty weapons. Jessica Lovering is looking at how the U.S. used to use and could in the future use nuclear exports to build relationships internationally to influence the safety and security regime. The concern now is that when you have countries like Russia and China, who are big nuclear exporters, they might not be as strict about some standards and might be willing to give enrichment or reprocessing technology to a country as an incentive to work with them. Lovering is looking for ways to enhance the U.S. position globally through more international collaboration on nuclear R&D and demonstration and prototypes to accelerate innovation and bring more countries into partnerships. Many countries in Africa are very interested in nuclear, since there is not a lot of coal and there is huge demand for electricity. Allowing everyone Western levels of human development and standard of living, such as agriculture modernization, requires a lot more energy and technology than they currently have. Nuclear power also provides energy security. China operates the second largest uranium mine in the world, which is in Africa. If the U.S. could have collaboration with other countries like South Korea to do a build in Africa, it would be much cooler model to see rather than having Russia or China come in and build a plant. Africa has a huge growth in engineers in many sub-Saharan countries and starting nuclear projects there is a great way to get more people in STEM, train people locally, and build out nuclear programs at universities. Getting the country and the local community engaged from the start has a higher likelihood of being successful.
29:35 - Advanced Nuclear Regulations
Bret Kugelmass: Is Canada trying to push forward on some advanced reactor licensing?
Jessica Lovering: Chalk River, a nuclear site in Canada, is trying to bring more interest in people building prototypes or demonstrations there. They have a more flexible regulator that gives a lot more feedback in the licensing process, so it is attractive for companies because it helps them figure out what their uncertainties are and what they need to prove for regulating in other places. Canada is trying to make themselves attractive for advanced nuclear companies, and companies like Canada’s Terrestrial Energy and other U.S. firms are already taking advantage of it. Jessica Lovering is really interested in seeing how fast floating nuclear or offshore nuclear could go. It is more of a different business model than a different technology, but it may accelerate the process for countries that don’t have the infrastructure set up or don’t have the security regulation in place. She is also interested in microreactors or autonomous reactors, which are minimally staffed, and the opportunities opened up there. If microreactors can be commercialized sooner, the U.S. has to rethink how they regulate nuclear if there are going to be a lot more microreactors in a lot more places. Other advanced nuclear companies are trying to be built into the commercial electricity market, whereas microreactors could compete with diesel or solar and could be very disruptive.
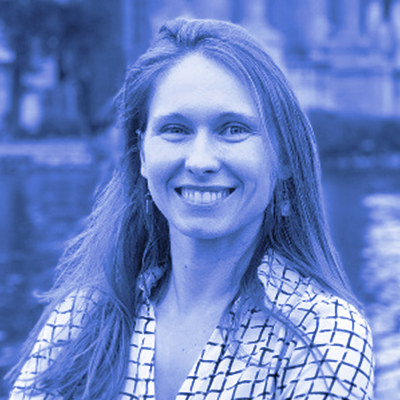
1) How Emma’s personal experiences abroad developed her interest in energy issues and her dual participation in both technical research and social advocacy
2) Developing nuclear fuel model simulator Cyclus and Emma’s efforts to evolve this model to incorporate a hybrid energy ecosystem as well
3) The role hybrid energy systems play in transforming the world
4) What happens when energy is made affordable
2:48 - Path to Nuclear
Bret Kugelmass: Tell me your story of how you came into nuclear.
Emma Redfoot: Emma Redfoot received her undergraduate degree in Environmental Studies from Lewis and Clark College in Portland, Oregon with the view that climate change is the issue of her generation. While she was in school, she spent six months living on a permaculture farm in Ecuador as an ecotourism intern. She also lived in Cusco, Peru for six months doing research on volunteer tourism, but didn’t see the scale of impact she wanted to see. Redfoot saw that energy was the fundamental thing that allowed people to empower themselves. There was a correlation between energy consumption and the increase of every standard of living, including women’s rights, longevity, health care accessibility, and education accessibility. At this point, Redfoot knew she wanted to study energy, decided she wanted to be an engineer, and started researching different energy topics, leading to her entrance into the nuclear engineering program at the University of Idaho.
7:09 - Nuclear Advocacy
Bret Kugelmass: Now that you are actively engaged in the nuclear space, where does your research take you?
Emma Redfoot: When Emma Redfoot visited the University of Idaho, she had the opportunity to meet with Dr. Shannon Bragg-Sitton. At the time, Redfoot was living in San Luis Obispo, California, home to Diablo Canyon Nuclear Power Plant. Before they announced the closure, Redfoot met up with Mothers for Nuclear, an advocacy group comprised of environmentalists focused on telling stories of why they think nuclear is important for the environment. She participated in an effort to go door-to-door and get people to sign a petition to keep Diablo Canyon open and a nuclear advocacy march in San Francisco. After moving to Idaho, Redfoot went to another march organized by Environmental Progress in Chicago, where she noticed that there were a lot of students present. Redfoot wanted to organize the student voices and open it to people who had different reasons for advocating for nuclear, not just environmental. Redfoot wants to see people pull themselves out of poverty with the availability of energy.
14:02 - Nuclear Renewable Hybrid Energy Systems
Bret Kugelmass: What’s your main focus of research at school?
Emma Redfoot: Emma Redfoot’s main focus of research at the University of Idaho is on nuclear renewable hybrid energy systems, led by Dr. Shannon Bragg-Sitton. The University of Idaho is in Moscow, Idaho, but the nuclear program is in Idaho Falls. The Center for Advanced Energy Studies combines Idaho National Lab, University of Idaho, Idaho State University, Boise State University, and the University of Wyoming. Redfoot originally got really excited about advanced reactors, but realized working on hybrid energy systems allows existing nuclear to be competitive with natural gas in cutting edge ways and decarbonize other industries besides electricity. Emma Redfoot has been working on developing a model in the nuclear fuel cycle simulator, Cyclus. This simulator goes through the whole fuel cycle, from mining, enrichment, fuel fabrication, through the reactor, and onto spent fuel to show different tactics for the industry based on different goals. Different people can develop archetypes and facilities within the program to try out a different form of storage with new characteristics or based on region. Cyclus is very flexible and fundamentally passes material between different facilities that are bidding on those resources. This program would create an incredibly dispatchable source of energy and would decarbonize industrial processes, potentially at a lower price. It is hard to find good metrics to define energy. Even with levelized cost of energy, there are many different values brought in to compare research. FERC is looking at reliability, EPRI is working on reliability, and emissions should also be valued. These factors are not always included in levelized cost of energy. Redfoot suggested one risk assessment approach called analytic hierarchy process, which allows one-to-one comparisons of things, such as whether desalination is safer than hydrogen production with an attached value. Certain characteristics are considered, such as safety, grid reliability, and profitability, and weighed by metric, allowing one-to-one comparisons.
24:26 - How to Make Nuclear Competitive
Bret Kugelmass: Where do you see your nuclear hybrid energy system research going?
Emma Redfoot: Cyclus is a very flexible, open source tool written in C++ that has a growing user base in nuclear. The research being done at the National Labs, the Modelica model, has a thorough model of hybrid energy systems overall. Modelica has a series of differential equations that controls the fluid flow and is an open source language specifically developed for dynamic systems. It can thoroughly model each of the components and look at the thermohydraulics within it, which is something not built into Cyclus. Hybrid energy systems have the potential to make a big impact within nuclear. A big way to make nuclear energy competitive is to put a cost on carbon, which is currently a huge externality not taken into account. This can broaden nuclear’s product base so it is more stable, since it won’t be selling just electricity, but another product as well, heat. It’s hard not to be excited about hydrogen as a fuel source and uses for metals like titanium. Emma Redfoot’s advocacy that she does is almost all surrounding support of existing reactors. The current fleet already produces 60% of the emissions-free electricity in the U.S. Keeping the existing industry alive can allow for advanced reactors to come into play. When nuclear reactors are close in the U.S., they are replaced by fossil fuel plants. Redfoot wanted to address energy poverty and light water reactor technology allows people to get access to energy without burning coal. Most people want to know they are leaving a better world for their kids. Nuclear advocacy is a long conversation every time and Redfoot aims to make nuclear more graspable for other people.
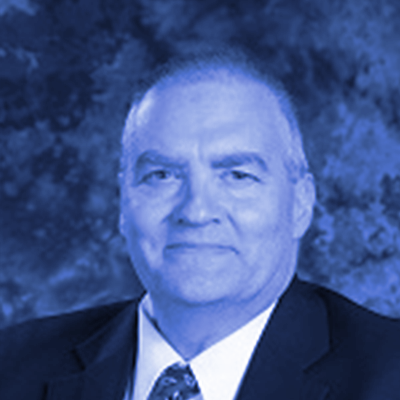
1) What it’s like to live in the community around and to work at a Nuclear Power plant
2) His work with the Light Water Reactor sustainability program and how they extend the life of the existing fleet
3) The role that Small Modular Reactors play in the future of the nuclear power industry
4) INL’s role in hosting a demonstration version of this technology and others
2:26 - Making Fuel for Boiling Water Reactors
Bret Kugelmass: How did you get involved in the nuclear space?
George Griffith: George Griffith studied nuclear engineering at Iowa State University and spent a summer working at Argonne National Lab in Chicago, where he was engaged with how to design things and tools used to make things. While at Argonne, Griffith worked at a pulse neutron source, which uses an accelerator to hit a heavy metal target to produce neutrons for scientific use. This led Griffith to pursue a PhD at North Carolina State University, focusing on computational methods and Monte Carlo. After finishing his doctorate, Griffith spent a couple years at Los Alamos National Lab before hiring on with General Electric where he started building nuclear fuel and was in charge of safety and quality for nuclear instruments. Uranium hexafluoride is heated up and turned into a gas, then converted into uranium dioxide, which is the material that goes inside the fuel rods. These are shaped into cylinders, loaded into cladding, welded up, and placed into bundles. This fuel was used for boiling water reactors (BWR), and had lots of enrichments and levels of poison to make sure they operate well in a boiling water environment. In a BWR, water comes in solid at the bottom of the reactor and heats up and turns into steam at the top of the reactor. This great change in density creates lots of fissions at the bottom and less as you get to the top. Letting that run without control creates an uneven distribution of power. Poison is added differently at the top and bottom of the core to control the power. The poison is a very small quantity of an element that wants to absorb the neutrons very aggressively.
7:06 - Industry Experience at Cooper Nuclear
Bret Kugelmass: What did you do after making fuel at General Electric?
George Griffith: After making fuel, George Griffith transitioned into working on design of reactors, specifically the design of the fuel, poisons, and enrichments. Griffith then moved into industry to work as a reactor engineer at Cooper Nuclear in southeast Nebraska. Communities appreciate the value the nuclear plant adds to the economy. As an engineer, Griffith would find out what happened at the plant overnight, perform triage on issues that came up, and work on longer term projects like reloading and maintenance. At this time, the reloading process what approximately 30 days that started with turning the reactor off, scramming it, and cooling it down. The reactor head is removed and the core is disassembled to access the fuel. A gantry crane is used to reach approximately 80 feet down. During reloading, there is usually double the staff on-site; at Cooper Nuclear, there were around 2,000 people on-site. Maintenance is also performed during the reloading outage, such as replacing turbines or generators. The plant had an outage center that monitored all activities during the outage and determined how the schedule was changing. During outages, staff usually puts in 12 hour days.
13:50 - Reactor Sustainability Program at INL
Bret Kugelmass: What happened after you worked at Cooper Nuclear plant?
George Griffith: After working at Cooper Nuclear, George Griffith moved to Idaho National Lab to work with the Light Water Reactor Sustainability (LWRS) program. The U.S. has around 100 light water reactors and these plants are reaching the point to be licensed from 40 to 60 years and a relicensing from 60 to 80 years is under consideration. The Nuclear Regulatory Commission (NRC) considers things like whether components are wearing out and the overall reliability. Some large questions remain, such as whether the steel in the vessel has changed with exposure to hot water, corrosion, and gamma rays over a span of 60 years. Instrumentation and nuclear fuels are also considered whether plants can operate safely and efficiently. They must also consider than standards, costs, and the size of plant security forces have gone up.
17:19 - Idaho National Lab Projects
Bret Kugelmass: What other work have you done at Idaho National Labs?
George Griffith: George Griffith worked with Idaho National Lab’s (INL) Advanced Test Reactor (ATR), the largest test reactor in the U.S. Experiments are placed in the ATR to prove that materials will last, fuels have been designed properly, and instrumentation can be tested in new radiation. INL’s primary customer at ATR is the U.S. Navy, but is also widely used by universities and companies to test their concepts. Their fuel cycles are around 80-90 days, so they are constantly in outage and designing cores. Griffith also did some work with advanced manufacturing, such as 3D printing of titanium for nuclear applications. Individual pellets have been made out of one homogeneous material, but they are looking into print pellets with different layers, which would allow for variations in enrichments, poisons, and structures that help heat escape. Accident tolerant fuels that result in less stress on the system if there were to be an incident at the reactor. The current fuel being used has been developed for 50 years, so it is a challenge to replace an optimized fuel with a new fuel that is better in every way.
21:43 - Current State of Small Modular Reactor Technology
Bret Kugelmass: What is an SMR?
George Griffith: An SMR is a small modular reactor, defined by the International Atomic Energy Agency (IAEA) as a reactor less than 300 MW. A typical reactor in the U.S. is around 1,000-1,200 MW. Two of these reactors are currently being built in the U.S. South, and two other projects are on hold. To build one of these projects requires multiple billions of dollars. The size of the project puts utilities at risk since it would take up a significant percentage of their industry. The idea of SMR’s is to scale nuclear reactors down. Traditional reactors are built on-site and require steel, concrete, and components to be brought in during construction. The SMR concept is to be built in a factory, which would take a lot of the economic risk to a place where it can be more easily controlled. NuScale is the most advanced SMR design team in the U.S. right now. They are designing a 50 MW water-cooled and water-moderated reactor that can be built in a factory. NuScale is also considering how to make their plants hypersafe, in the sense that, if there was an incident, operators would take their hands off the controls and leave the reactor and the reactor would be alright. NuScale has been working on their development for about 15 years. Building the large plants on-site with contractors has challenges and risk, since there have not been many reactors built in the U.S. SMR’s aims to fix the economics, the deployment issues, and build issues. Each designer comes up with a different solution to that challenge. Others are using advanced reactor designs, such as molten salts and molten leads. NuScale is working with the Nuclear Regulatory Commission (NRC) towards their application. Idaho National Lab (INL) may host the first nuclear power plant using NuScale technology. Utah Association of Municipal Power Systems (UAMPS) is looking to own the reactor at the INL site to help them supply energy into the system. The benefit of having this UAMPS site at INL is that INL knows the area, including seismic, water, animals, etc. since they have been in Idaho for over 50 years. George Griffiths translates between different teams, including siting, engineers, and others. In the next couple of years, the plant will be under construction at INL.
33:03 - How Economics Drive Nuclear Technology
Bret Kugelmass: How do you see nuclear technology evolving over time?
George Griffith: It seems that the really large plants are particularly difficult to build. If the four plants in the South can be successfully pulled off and proved that they are economically viable for the companies that bought them and the communities that supported them, there will probably be a few more. If those plants don’t deploy, the U.S. is left with small modular reactor (SMR’s) concepts. While large plants need specialized transmission resources, SMR’s allows for deployment in different markets and replacement of coal plants, which are typically smaller. Everybody is waiting for the first SMR successful deployment to prove it works and can be done.
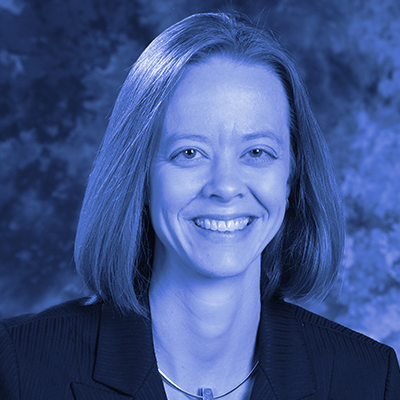
1) Her entrance to the Nuclear field at an incredibly young age
2) An overview of space nuclear reactors
3) Nuclear energy as an Industrial heat source and how it fits into broader energy ecosystem
4) Laboratory scale model validation of the worlds future integrated energy systems
2:52 - Space Nuclear Reactors
Bret Kugelmass: How did you get into the nuclear space?
Shannon Bragg-Sitton: Shannon Bragg-Sitton grew up in Albuquerque, New Mexico, located near Kirtland Air Force Base, Sandia National Lab, and Los Alamos National Lab. Her first job as a high school student was working on space nuclear reactors. In the early 1990’s, the U.S. purchased six non-nuclear electrically heated test units for space reactors from the former Soviet Union. They were brought over to the U.S. to configure, test, and see if there was a desire them in the U.S. space program. Bragg-Sitton, fascinated by the technology and by space, worked alongside many Russians and interpreters on the space reactors. This inspired her to enter a Bachelor's program in nuclear engineering. These space reactors used the reactor to produce electricity, which then drove electric propulsion systems. Other systems were nuclear thermal propulsion systems, in which the reactor coolant, typically hydrogen, was used as the propellant. These types of power sources open up opportunities to space travel, exploration, and discovery that is much beyond what current systems can do. In and after graduate school, Bragg-Sitton focused on nuclear electric propulsion systems with NASA. When something really difficult, like space travel, is combined with something challenging, like nuclear, it takes time to get projects through to completion.
7:16 - Contributions to National Lab Programs
Bret Kugelmass: What did you do after your time at NASA?
Shannon Bragg-Sitton: When Shannon Bragg-Sitton worked at NASA, she was actually an employee of Los Alamos National Lab on loan to NASA. Her alma mater, Texas A&M, asked her to come back as faculty in the nuclear engineering program. Texas A&M has the largest undergraduate population in nuclear engineering and is the only university to operate two research reactors. Bragg-Sitton spent three years at Texas A&M developing programs and classes in space nuclear systems and plasma physics. She decided to return to the National Laboratory to get back into the fundamental aspects of developing space nuclear systems. In her current position, Bragg-Sitton still maintained ties with several universities and working with students on committees and PhD projects, but was more in a position where she could help define programs and establish the way forward for nuclear energy. In the past seven years at the National Lab, she has worked on advanced nuclear fuel, advanced energy systems, small modular reactors, advanced reactors, and ways in which those systems can supply the nation’s energy needs in a different way. Light water reactors produce electricity and provide resilient baseload power, but throw away a lot of thermal energy. Baseload supply power is being stressed by outside factors of renewable energy and natural gas and needs to operate more flexibility or modify their power to meet the volatility of renewable energy. Solar and wind energy are non-dispatchable energy sources, since they are only available when the sun and wind are available. Energy storage helps protract this out, but not in the long term and seasonal storage. A majority of the solar and wind resources are in specialized locations. Intermittency and transmission are two major challenges in the electricity market. Emissions coming from resources used for energy is also a consideration. However, electricity is only one piece of the puzzle. The energy sectors include electricity, industrial energy, transportation, and residential heating. Some industrial processes burn carbon resources to create the heat necessary to drive these processes, such as in plastic production.
15:31 - Nuclear Renewable Hybrid Energy Systems
Bret Kugelmass: What is your Nuclear Renewable Hybrid Energy Systems program?
Shannon Bragg-Sitton: Shannon Bragg-Sitton wants nuclear power reactors to work in coordination with renewable technologies coming online in certain regions. Nuclear can technically modify the power output within certain constraints. A reactor operates best when it is at its nominal operating power level running steady state. Instead of changing that, some energy produced could go to the electricity sector and the excess heat not needed for electricity can be sent to other things, such as industrial processes or district heating. Waste heat can be used in district heating to send steam to the region. Higher quality heat can drive industrial processes and water desalination. The most commercially available option for water desalination is reverse osmosis, which is driven solely by electricity. Bragg-Sitton’s program is managed under the Office of Nuclear Energy in the Department of Energy. Her program has a collaborative sister program in the Office of Energy Efficiency and Renewable Energy, whose focus in hybrid energy systems is in hydrogen. Hydrogen at Scale looks at large-scale production of hydrogen through the use of clean energy sources. Hydrogen can be used as a transportation means in fuel cell vehicles and is an entry into other industrial processes, such as upgrading biofuels. Bragg-Sitton’s program looks at how to integrate hydrogen production into a nuclear system, such as with low temperature electrolysis or high temperature electrolysis and how they can be operated flexibly. She started looking at preliminary analysis using simplified models, then looking into more dynamic and higher fidelity models to determine how to move energy around. Each subsystem has different response times and power changes. A control system architecture must allow this energy to be lined up and balanced, but models must be validated. Models are moved into a laboratory where systems can be put together with controlled heat sources to mimic the behavior and thermal energy input. In the integrated systems laboratory, there is a dynamic load and acts like users on the grid to see the variation of electricity demand. Actual solar panels and wind turbines are integrated into the lab and computer systems represent a microgrid. Real time digital simulators emulate the power systems on the grid. These pieces are physically put together for hardware in-the-loop testing to understand how the systems behave.
25:33 - Implementation of Hybrid Energy Systems
Bret Kugelmass: As you progress your research, who are the eventual stakeholders for this technology that might implement it in the real world?
Shannon Bragg-Sitton: When Shannon Bragg-Sitton first started the program, the focus was on advanced reactor technologies which operate at higher temperatures than light water reactors, bringing higher efficiency. The program looked for benefits at lower temperature light water reactors and started getting interest from the current fleet of light water reactors which are being operated by utilities that are currently seeing economic challenges for their reactors. Bragg-Sitton is working with a number of operating utilities at existing plants and has done a number of initial analyses for advanced reactor developers and developers of light water small modular reactors to look at the feasibility of the systems. The user community, such as fuel cell producers, are also brought in to talk about fabrication and distribution using hydrogen. Bragg-Sitton maintains a utility advisory committee for her Hybrid Energy Systems program, relying on operators to share how they’d realistically accomplish integration in a current operating reactor, without impacts to safety systems, regulations, and licenses. The National Laboratory is coming up with the ideas and working with industry directly for them to advise on strategies for implementation.
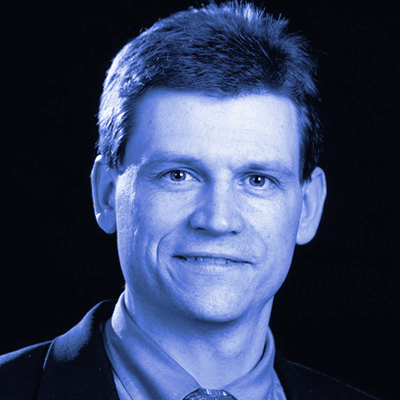
1) How Hans became interested in nuclear energy
2) How to lay the foundation for commercializing new technologies
3) The role INL plays as an independent auditor and fuel qualifier
4) The difference between pebblebed and prismatic reactors
2:32 - Introduction to Nuclear
Bret Kugelmass: How did you get involved in the nuclear space?
Hans Gougar: Hans Gougar studied physics in his undergraduate studies at the University of Wisconsin and started out teaching high school physics. Five years in, while teaching in London, England, Gougar met his wife-to-be, Mary Lou, and they found a common interest in the nuclear curriculum. Hans Gougar ended up at Penn State where he studied nuclear engineering with a focus on advanced nuclear power. Nuclear energy is the manipulation of matter on a fundamental, nuclear level to generate electricity, perform medical procedures, and power water and space vehicles. It has only been a little over 100 years since humans were able to probe the interior of the atom, the nucleus. While at Penn State, Mary Lou obtained a fellowship to work on a dissertation that required her to work in Idaho at Argonne West and Hans got a job at Idaho National Lab. He started out working on the Advanced Test Reactor, then was asked to help out on a new pebble bed project at the Laboratory. The U.S. had seen the pebble bed work going on in South Africa and there seemed to be a resurgence in interest in high temperature reactors around the world. Exelon, a utility in Eastern U.S., committed to investing in and building this technology. The lab said they would look into the technology and see how they could help out. An internal project was set up in conjunction with MIT.
7:51 - Pebble Bed Reactors
Bret Kugelmass: What is pebble bed technology and how do high temperature reactors work?
Hans Gougar: Pebble bed reactors run at the highest temperature of any other type of power reactor and operates between 700-1,000 degrees Celsius outlet temperature. The high temperature is allowed by the structure of the reactor. Instead of having oxide fuel rods in metal cans with water flowing through it, as in light water reactors, high temperature reactors have very small, millimeter size uranium oxide particles that are coated with various layers of silicon carbide, high density carbon, and other materials. Thousand or millions of these particles are encased in either a graphite pebble, about the size of a billiard ball, or a compact, cylindrical-shaped element encased in larger blocks. Like a gumball machine, a cavity is filled with the pebbles, which contain thousands of fuel particles. The coatings on the pebbles are highly robust and it is very difficult to break them in the conditions inside the reactor. A uranium oxide or carbide kernel is surrounded by a low density, spongy buffer material that can absorb and hold onto fission products, a high density layer of pyrolytic carbon, silicon carbide, the main fission product retaining barrier, and finally one more layer of pyrolytic carbon. The multilayer system is very effective at keeping nuclear stuff inside. When this is embedded in graphite, it creates another barrier, but any excess heat that may be generated during an off-normal incident gets absorbed by the graphite and transmitted away out of the core without harming or allowing the fuel particles inside to undergo any damage. Helium is used as the coolant in the pebble bed system since it doesn’t corrode anything and is very inert neutronically and chemically. The helium carries the heat away where you can do something useful with it, whether that is high efficiency electricity generation or industrial processes that are driven by heat.
13:33 - Resurgence of Pebble Bed Technology
Bret Kugelmass: Worldwide, where are we now with pebble bed technology?
Hans Gougar: Pebble bed technology was first developed in the 1970’s and 1980’s in Germany. In the U.S., prismatic high temperatures reactors were built, including Fort St. Vrain. The technology faded out and was competing with light water technology that had the major support from the government and the industry at the time. In the 1990’s, interest surged after the accidents at Three Mile Island and Chernobyl. China and Japan had just finished building small, engineering scale reactors that are still operable today and used for experimental data collection. The Japanese have plans to use their test reactor to explore power conversion systems like a gas turbine or hydrogen production, but they are not meant to generate revenue or electricity. A number of scientists in South Africa in the 1990’s decided they could adopt this technology and was right for their market and mining industry. They started a government-funded project called the Pebble Bed Modular Reactor (PBMR). Hans and Mary Lou Gougar decided to take the opportunity to see the industry from a different perspective and spent a little over a year helping out at PBMR in South Africa.
17:56 - Pebble Bed Research at INL
Bret Kugelmass: When did you return to Idaho National Lab and what has been their focus since then?
Hans Gougar: Hans Gougar left the Pebble Bed Modular Reactor (PBMR) project in South Africa and returned to Idaho National Lab (INL) in 2009. INL has four main research areas within the high temperature reactor project, originally called the Next Generation Nuclear Plant. The main focus was on improving the particle and standing up a commercial provider for the fuel, since the entire fuel infrastructure in the U.S. was focused on the light water reactor. INL is looking at new grades of graphite, qualifying them, and understanding how they behave inside the reactor environment. There are metallic components inside the vessel itself and the metals that are useful or qualified for use in nuclear applications cannot easily withstand those higher temperatures, so new metals need to be found, qualified, and made available to the industry. INL also works on simulation design and analysis methods that are needed to license such a machine. The regulator and investors must be convinced that the reactor temperature is known throughout and how much fission is taking place. They also want to know about its behavior if something goes wrong and off-site cooling is lost. In the early days, German pebble bed reactors used loss of coolant as a shut down mechanism. The primary coolers and blowers would be turned off, increasing the temperature a little bit, but by natural feedback mechanisms, would turn itself off and cool itself down. The affinity for neutrons by the nuclear fuel is very temperature sensitive. As you raise the temperature of the fuel, it is less likely to absorb neutrons and cause fission.
22:39 - Qualification Process for New Fuels and Materials
Bret Kugelmass: What are some challenges of pebble bed technology?
Hans Gougar: Pebble bed reactors face the same challenge of cost of other nuclear power plants. There is no constant feedback and product improvement, limiting the opportunity to learn and innovate. Reactors must go through the same licensing process and come up with the same supply chain for fuel and components. It is a multibillion dollar adventure and investors are hesitant to get in. Hans Gougar and the Idaho National Lab (INL) are working with the Nuclear Regulatory Commission (NRC) to modify the regulations so they are not light water specific, but are more generic in nature. The NRC must be able to understand the technology first and then define the appropriate limits and operating regimes for the system. INL provides an independent auditing or confirmation expertise to the NRC and startup companies. The bulk of INL’s work has been on basic fuel and material qualifications. INL fabricates the fuel, puts it in the test reactor, and burns it to a crisp to understand how it behaves and how well it retains the fission products. This data is supplied to the vendors and the regulator. In the U.S., the primary configuration is a prismatic reactor. Instead of a gumball machine setup, the reactor has cylindrical fuel elements, about the size of a pinky finger. These cylinders are stacked inside of a larger graphite blocks stacked together and helium is blown through them.
27:56 - Future Deployment of High Temperature Nuclear Plants
Bret Kugelmass: Where do you see the future work of your work, INL, and pebble bed nuclear technology going?
Hans Gougar: Pebble bed technology is poised for major deployment, but the U.S. must relearn how to build nuclear power plants inexpensively. These plants are highly engineered, complex machines. To build one on-time and on-schedule according to your designer’s specs is a tremendous architectural engineering effort. Costs in the U.S. have gone up in the areas of engineering procurement construction (EPC), labor, and supply chain. If you can build a machine that is inherently safe, it allows to you have a lower cost machine, but it is still very complex and expensive. People are concerned about carbon footprints and fossil fuels. Natural gas is keeping our economy going, but eventually prices might spike again depending on local supply conditions. If gas prices get around $8-9 per million BTU, a high temperature reactor can compete in both the electricity and process heat marketplace with natural gas.
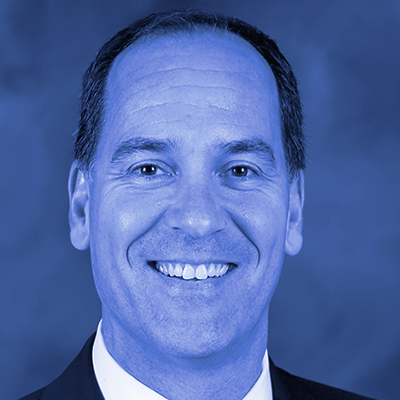
1) John’s early experience at Oak Ridge National Labs and expertise on understanding how radiation moves through matter
2) The basics of spent fuel management and the status of Yucca Mountain.
3) The five different divisions that fall under the Nuclear Science Directorate
4) The concept of transients and how INL tests them
Q - Early Exposure to Nuclear at National Labs
Bret Kugelmass: How did you find your way into a nuclear profession?
John Wagner: John Wagner bounced around the different engineering departments at the University of Missouri-Rolla before getting excited about nuclear physics and engineering. During his undergrad, Wagner spent a summer at Oak Ridge National Laboratory working on the High Flux Isotope Reactor. Between undergrad and graduate school, went to Los Alamos National Laboratory where he got involved in Monte Carlo radiation transport methods. That summer was a career changing experience and took him to graduate school at Penn State to receive his Master’s and PhD with a focus on Monte Carlo. If you want to know a person’s dose rate of radiation exposure and design the safety of a system, such as shielding, you have to understand how radiation moves through matter. The principle ionizing radiation protected against are neutrons and gammas. Radiation transport is a computational or analytical tool for simulating the dose rate in different parts of a system. Monte Carlo follows individual neutrons and, based on the statistics, infers the average behavior of all the neutrons in a system. Wagner combined Monte Carlo radiation transport methods with traditional deterministic transport methods into hybrid transport method. After grad school, John Wagner joined Holtec International working on criticality safety analyses for spent fuel rocks, which are steel rocks that sit in a spent fuel pool. When fuel is initially taken out of the reactor core, it sits in pools to cool and water provides shielding from radiation. From there it is transferred into dry storage casks. Dry casks are certified for 20 years for commercial spent nuclear fuel. As on-site storage in the industry grew, Holtec had a market to put higher density racks in the pool to increase the capability of the pools. The Nuclear Regulatory Commission (NRC) worked with the industry in terms of what they saw as requirements for ensuring the safety and continued performance of the systems, allowing extension of the licenses up to 40 years. The industry has active aging management programs in which people inspect the canisters and casks to ensure they are continuing to perform and look for signs of degradation.
13:12 Q - Storage Options for Spent Fuel
Bret Kugelmass: After Holtec, where did you go?
John Wagner: After a couple years at Holtec, John Wagner was offered the opportunity to return to Oak Ridge National Laboratory (ORNL) and get back into his radiation transport work. A few different codes being used around the world were developed at ORNL using those methods. These codes are pieces of software that are executed to solve a problem. These methods enabled calculations that were previously computationally prohibitive. While at ORNL, Wagner got involved with the Nuclear Regulatory Commission (NRC), ultimately working on the proposed repository at Yucca Mountain. John Wagner was part of the Yucca Mountain team that submitted the license application in June 2008. The NRC has reviewed it and issued safety reports that are favorable. The program has since been defunded, but from a technical standpoint, the repository is very viable. There are efforts on both sides of the political aisle to support or not support Yucca Mountain. The local community is supportive and wants the license to go all the way to go through the system and get executed if it is safe. Interim storage is needed, but the industry struggles with the social and political aspects of the technology. The dose rates at the storage casks are very, very small. The Nuclear Regulatory Commission (NRC) focuses on the site boundary does, approximately one-third of what a cross-country airplane. The people that would be close to the casks may be security, maintenance, or aging management personnel. Due to radiation decay, the radiation dose rates get lower.
20:26 Q - Idaho National Laboratory
Bret Kugelmass: How did you get involved at Idaho National Laboratory (INL)?
John Wagner: Idaho National Laboratory (INL) has complex tools and facilities for the Advanced Test Reactor, such as the fuel examination facility and the irradiated materials characterization lab. INL had all the ingredients and abilities on-site to make different fuel types and materials. Test fuels go through pre-irradiation characterization, get sent to the Advanced Test Reactor, go back to the hot fuels examination facility, and get sample prepped to go into different microscopes. By understanding how fuels and materials behave in a radiation environment allows scientists to inform their computational models. Research is pushing in the direction of getting data to develop predictive capabilities so that experiments are used to confirm what codes are telling us. Experiments are expensive and time consuming. John Wagner runs a directorate which is made up of five different divisions, which are Domestic Programs, Nuclear Fuels and Materials, Fuel Cycle Technology, Nuclear Systems and Analysis, and Regulatory Safety. The Regulatory Safety division informs current or new regulations and helps them understand technical bases for different regulations.
25:47 Q - Advanced Reactor Research
Bret Kugelmass: Tell me about some of the advanced reactor work going on at Idaho National Lab.
John Wagner: There is a lot of external and private interest in advanced reactors. The nuclear community has transformed in the last 5-10 years from a few very big companies, like Westinghouse, to a lot of small startup companies that are focused on advanced reactor designs. Some advanced reactor designs include high temperature gas reactors and fast reactors. The Transient Reactor Test Facility (TREAT) shut down in 1994 and initially went critical in November, meaning steady state, and that reactor is intended to be a pulse or a transient reactor. A transient is a spike in power. A normal commercial reactor does not want transients, but instead wants to run steady state. In the event you did have a power spike, you need to understand how the fuel behaves. If your fuel doesn’t perform well under that environment, you may have to replace the assembly or it may have a big impact on the reactor core and is very important for licensing. Light water reactors use water to cool and moderate the neutrons. Advanced reactors are looking at different concepts that are more passively safe. A less mature class of advanced reactors is molten salt reactors. It is the only design that has the fuel in a liquid form. This offers the ability to continually operate and replenish salt. Fast reactors, fast spectrum neutrons, are better suited for fuel recycling. The Experimental Breeding Reactor 2 (EBR-2) was linked with a fuel conditioning facility and demonstrated full fuel recycling. Fast neutrons turn uranium-238 into plutonium-239 which is a fissile isotope. All the light water reactors in the U.S. have uranium-235 as the core ingredient in their fuel, which releases on average 2.5 neutrons out which go out to have another chain reaction. Plutonium-239 production was done at the Hanford site to create nuclear weapons. In civil applications, you can get to a point in which you are making more fissile isotopes than you are burning, creating an unlimited energy source. John Wagner got into nuclear energy from the standpoint of abundant, reliable clean energy. Energy conservation is helpful, but is not the answer to energy poverty. Nuclear is effectively an infinite source of energy. The facilities at Idaho National Laboratory drew Wagner in, but he was also attracted to the focus on nuclear energy.
36:22 Q - New Fuel Development at INL
Bret Kugelmass: What are some things you see coming out of the Idaho National Lab in the future?
John Wagner: The number of nuclear reactors in the U.S. is declining and there are a lot of challenges in cost, especially compared to wind, solar, and gas. Idaho National Lab (INL) wants to help nuclear plants reduce their operating cost. At the end of the day, we want to see the nation use diverse clean energy sources, but there is a commercial market and they must be cost competitive. Wagner is excited about the small startup companies in advanced nuclear and the push on innovation in technology, methods, and approaches. Accident tolerant fuels are more robust in the event of an off-normal situation, such as Fukushima, and provide as much time as possible to mitigate a situation. This fuel has the potential to be less expensive and more efficient, which will help with reactor operating cost. INL is getting ready to put three different concepts into the Advanced Test Reactor later in 2018. They are making uranium silicide fuel at the Materials and Fuels Complex which is targeted to go into a commercial reactor in 2019. One of the challenges with nuclear is how long it takes and all parts of the equation need to be more efficient. When designing new materials, it must be made and you must understand the material. Then it is exposed to radiation, high temperatures, or corrosion environments, whichever end use environment it will be exposed to. Higher flux research reactors are used to accelerate the process, but it still takes time. After taking it out of a reactor, it is both very radioactive and hot. By the time you get data, there is a chance it may not be the data you need. Innovation must be faster and rely more on modeling and simulation and get creative about how to create environments that can accelerate testing.
41:22 Q - Economic Uncertainty of Nuclear
Bret Kugelmass: What’s your vision for the future of nuclear energy?
John Wagner: A typical nuclear energy plant in the U.S. is very big, on the order of one gigawatt electric, take 8-10 years build, and are many billions of dollars. This is a big hurdle for a utility to commit to. The uncertainties and risks are significant. Right now, the economics drive them to putting natural gas or other sources on. Research at Idaho National Laboratory (INL) fits in three buckets: helping the existing fleet stay viable, advanced reactors, and the fuel cycle. Microreactors are very small reactors that could be built in a factory. INL is working with private companies and intend to demonstrate a microreactor on-site by 2021. Its characteristics are intrinsically safe and its systems are based on technologies that have been developed over decades. Interest for microreactors is in remote communities and special purpose type applications, such as the Department of Defense. INL wants to demonstrate that microreactors are possible and open up the future for nuclear in a small, modular way.
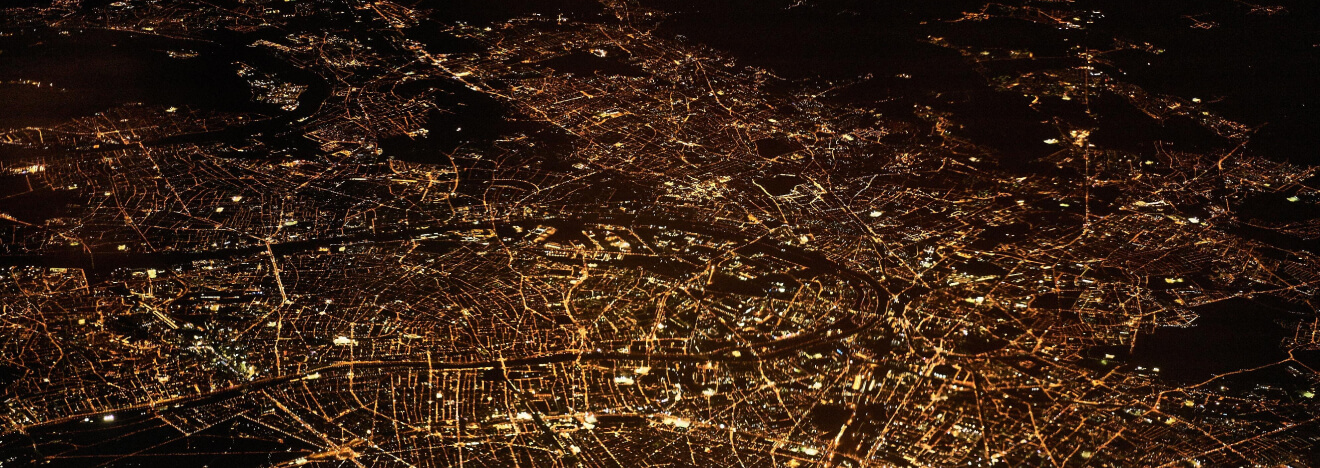
Sign up for our newsletter
No results found
Please try different keywords