TITANS OF NUCLEAR
A podcast featuring interviews with experts across technology, industry, economics, policy and more.
Latest Episode

1) The beginning of Brian’s career and his time in the United States Navy as a diver, as well as what drew him to engineering and nuclear
2) Brian’s initial journey to Oregon State and all of the research projects he’s had a hand in since then
3) Fostering a passion for nuclear in the next generation of nuclear engineers and why the researchers as just as important as the research itself
4) What challenges and successes the nuclear industry will face in the coming years and how to form your individual opinion on nuclear energy

1) Kathryn’s background in nuclear engineering and personal journey in the nuclear industry
2) The Advanced Fuel Cycle Initiative aka The Fuel Cycle R&D Program and Kathryn’s work optimizing the backend of the fuel cycle
3) Canada Nuclear Lab’s mission to promote SMRs
4) Canada Nuclear Lab’s research surrounding radiation and targeted alpha therapy and their potential impact on the industry at large
1 - Early International Nuclear Studies
Bret Kugelmass: Tell me about your early interest in nuclear.
Kathryn McCarthy: Kathryn McCarthy grew up around the San Francisco Bay area since her father worked at Livermore Lab, one of the weapons labs that happens nuclear material. This exposed her early on to many engineers and physicists. In high school, McCarthy planned to pursue music, but a passionate high school physics teacher inspired her to study nuclear engineering at the University of Arizona. One of her professors was Norman Hilberry who was the axman in the first Chicago Pile Experiment. This was one of the original critical experiments which proved that fission could be controlled. Hilberry mentored McCarthy during her time in Arizona and she eventually left to pursue her graduate degree in nuclear engineering at UCLA. At the time, there wasn’t much R&D in the fission world, so McCarthy’s graduate work was related to fusion, specifically liquid metal magnetohydrodynamics. She finished graduate school and worked abroad for six months in West Germany at the Kernforschungszentrum, which later became Karlsruhe Institute of Technology. McCarthy and her husband then went to the Soviet Union in 1989 and 1990 working on liquid metal magnetohydrodynamics.
2 - Path to Idaho National Laboratory
Bret Kugelmass: Where you in the Soviet Union during the collapse?
Kathryn McCarthy: In August 1989, Kathryn McCarthy and her husband were in Leningrad having dinner with some friends who worked at the French consulate. Her friends had heard that wall had come down in Germany, but didn’t know if it was true. Soviet news downplayed the event. McCarthy’s assignment ended in September 1990, just before the coup in December. Upon returning to the U.S., she took a position at the Idaho National Laboratory (INL) where she spent 25 years, starting out in the fusion safety program. A model was developed to look at safety analysis of the fusion machine to understand pressure drops and heat profiles, which fed into McCarthy’s research. She was also responsible for volatilization of materials experiments. When tokamaks are exposed to high heat, there may be an air ingress and some of the material can oxidize, creating dust or particulates. Temperatures in tokamaks are higher than the temperature of the sun. Liquid metal is a coolant, absorbs neutrons, and can take very high heat flux. McCarthy became a manager responsible for the fusion work and some of the fission work, eventually focusing on the advanced fuel cycle initiative. She was on the Department of Energy (DOE) Fusion Energy Sciences Advisory Committee (FESAC), allowing her to stay engaged in the fusion community.
3 - End of the Nuclear Fuel Cycle
Bret Kugelmass: At which point did you switch to the fuel cycle?
Kathryn McCarthy: Kathryn McCarthy switched to focus on the fuel cycle when a new contractor, Battelle, came into Idaho National Laboratory (INL). They wanted her to take over systems analysis for the advanced fuel cycle initiative. The focus at the time was on the back end of the fuel cycle, looking at disposal or recycling. There were other disposal options that came up other than Yucca Mountain, but the question was what would actually go into disposal. Frane recycles the plutonium, but the rest of it gets disposed of, which is where some of the high heat and radiotoxic material. Options for the end of the fuel cycle depend on which constraints are used, such as a constraint for never having pure plutonium separated in any streams. McCarthy looked at the whole system, including number and size of tanks, supply chain, and transportation. There are scientific solutions to spent fuel recycling, but the urgency argument is hard to make since there is a lot of uranium. Another argument is that humans should be good stewards of all resources for all generations to come, which is true, but loses the urgency. Because of process losses, there must always be some sort of long-term repository, but the size and number of these repositories can be minimized. Nuclear energy, science, and technology was always the core of INL.
4 - Canadian Nuclear Laboratories
Bret Kugelmass: What was the opportunity that resonated with you to join Canadian Nuclear Laboratories?
Kathryn McCarthy: Kathryn McCarthy took an opportunity with Canadian Nuclear Laboratories (CNL), which used to be a GOGO (government-owned, government-operated) entity called Atomic Energy Canada Limited (AECL). It was changed to a GOCO (government-owned, contractor-operated) so contractors could bring in more of a business-like sense in making decisions based on priorities and increasing the non-government work. CNL is a sister lab to Idaho National Laboratory (INL). McCarthy participated in rebuilding CNL, which had not gotten investments made over many years. When it was restructured to GOCO, the government provided R&D funding and, over the ten year contract, $1.2 billion dollars for infrastructure. One of the new buildings will be an advanced nuclear materials research center and some of the existing facilities are being revitalized. The Canadian government decided they were not going to get back into the business of designing reactors; AECL designed the CANDU reactor. The design for NuScale started as a LDRD (laboratory directed R&D) project that was done in conjunction with Oregon State University. There must be a marriage between the National Labs and the industry. The labs have great ideas, but sometimes practical implementation takes a discussion. Hydrogen is an energy carrier. Right now, the majority of hydrogen is produced from methane. There are better uses for methane and it produces greenhouse gases in hydrogen production.
5 - Small Modular Reactors in Canada
Bret Kugelmass: Would a hydrocarbon be broken up to produce hydrogen?
Kathryn McCarthy: Canadian Nuclear Laboratories (CNL) worked with Jacobs on a project in Toronto to look at converting the light rail to either electric or hydrogen, specifically related to safety. Under certain circumstances, there can be hydrogen explosions. At Fukushima, there were hydrogen explosions because there was an accumulation of hydrogen exposed to oxygen. Moving to hydrogen powered cars requireds a distribution system. Right now, CNL is looking at heavy transport such as trucks and trains, especially in the far northern regions that don’t have current gas infrastructure and investments must be made. Canada is an attractive place for small modular reactor (SMR) vendors is that there is a market of remote communities and mines which currently rely on diesel, currently estimated at 200-300 communities. Mines would be interested in an SMR on the order of 10-30 megawatts, but it must be able to compete with the cost of diesel, which is expensive. In 2018, CNL put out a process for applications to a site, which was partially based on a request for expressions of interest that had been put out in 2017. Canada has potential market opportunities before export. The Canadian regulator’s framework is technology-neutral, meaning it is very outcome-oriented compared to the process-oriented U.S. Nuclear Regulatory Commission (NRC).
6 - Biological Radiation Applications
Bret Kugelmass: Is there an alternative mechanism in Canada in which the national lab system could have a parallel license project for microreactors that might go on lab land?
Kathryn McCarthy: Canadian Nuclear Laboratories (CNL) is regulated by the Canadian regulator, as opposed to the U.S. National Labs. CNL has a goal to demonstrate a small modular reactor (SMR) by 2026. They worked backwards from that date and built in minimum timelines for the regulator. This requires a design that is close to ready to go to meet the 2026 goal, but the goal is still feasible. CNL received four applications to site for SMR’s and there are one to three additional applicants that plan to apply. CNL historically has done work on the biological effects of radiation and a large portion of the worldwide database for effects of low-level radiation was developed at CNL. This database shows that the linear no-threshold theory shouldn’t be used, but it’s difficult to undo something that has been in place for a long time. People look at medical radiation as different than nuclear energy radiation. Radiation is used to treat tumors, but there is a lot of collateral damage with treatment from outside the body. With alpha therapy, compounds could be attracted to the tumor and target the tumor specifically. The nuclear industry needs to do a better job with talking about things that get used in nuclear treatments and diagnostics. There are everyday uses for radiation that people don’t realize. Nuclear is essential to climate change mitigation, having clean air, and having affordable energy broadly available.
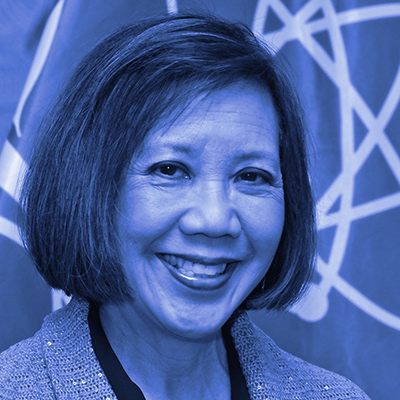
1) Janice Dunn Lee’s 40-year journey through nuclear from her position in Export Licensing at the NRC to that of Deputy Director General at the IAEA
2) Intricacies of international nuclear agreements including 123 agreements for nuclear cooperation between the US, Japan, and China
3) The importance of bilateral and multilateral cooperation agreements in helping countries learn the regulations, inspection processes, and licensing in other countries
4) Humanitarian aspects of nuclear and the IAEA’s work in human health including cancer therapy and ebola prevention
At this time we are still producing show notes for this episode. Please check back again at a future date.
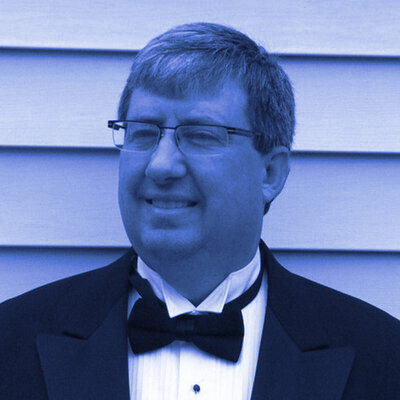
1) Ed’s background in the US Navy and his personal journey in the nuclear industry
2) Nuclear opponents and their negative effects on the construction and licensing processes
3) How the US nuclear industry is working to overcome supply chain challenges including replacing medium enriched uranium with plutonium
4) The plant Ed envisions including its total power output and the regulatory and financial hurdles involved
1 - Navy Nuclear Design
Bret Kugelmass: Tell me about your experiences in the Navy designing reactors.
Ed Pheil: In 1984, Ed Pheil started at the Knolls Atomic Power Lab training Navy students at the Kesselring Site prototypes. He then moved into design, spending the remainder of his career designing nuclear submarines, aircraft carriers, and for a time, spacecraft to go to Jupiter. Pheil supported all Navy reactors at the time and multiple new designs, such as Virginia-class and Ohio-class. The Navy utilizes pressurized water reactors (PWR), but has always been looking for something that would be more of an advanced reactor to provide better performance. However, changing to different fluids and different temperatures also requires fuel redesign, which was outside the Navy budget. Submarine reactors are much smaller than commercial reactors. They propel the ship and provide support for about 100 people. The commercial sector is interested in Navy nuclear personnel because they are used to startup, shutdown, and casualty drills on a regular basis, providing much more experience in variants. The Navy has two Naval nuclear laboratories: Bettis Atomic Power Lab and Knolls Atomic Power Lab. Teams at the labs work together on new design, maintenance of existing fleet, and decommissioning. Electric Boat, part of General Dynamics, and Huntington Ingalls run the shipyards where submarine building takes place. The Newport News Shipyard is involved in the AP-1000 construction. Electric Boat has the biggest experience in modular construction. Both companies have civilian arms that do work in the nuclear sector. Pheil spent a total of 32 years in the Navy.
2 - Reimagining the Nuclear Fuel Cycle
Bret Kugelmass: How many different types of reactors did you look at during your 32 years in the Navy?
Ed Pheil: During Ed Pheil’s 32 years in the Navy, he looked at about 20 different types of reactors, such as lead reactors, lithium reactors, molten salt reactors, and more. The future of energy is the uranium-plutonium cycle. All the spent fuel has 96% of uranium left in it; in reality, only 0.5% of the uranium extracted from the ground is being burned. All the depleted uranium is fuel too, in the right reactor. This requires a low cost fast reactor to close the fuel cycle. Elysium’s reactor is a molten salt fast reactor using chloride salt. The uranium is dissolved into the salt. Weapons-grade plutonium is diluted into spent nuclear fuel to make it non-weapons grade. This reduces the weapons risk while creating new fuel and eliminating spent nuclear fuel. This can be done with other fast reactors, but solid fuel is needed, which requires very clean uranium and plutonium. A liquid fuel doesn’t need to be cleaned up because there is only one step to convert it. While in the Navy, Pheil got bored of operating, starting, and maintaining water reactors. He knew the commercial industry had lost experience in building reactors and wanted to do something for the commercial industry to save nuclear power, leaving the Navy before he was eligible for retirement. Pheil’s undergraduate degree was in fusion and he reviewed that technology for practicality as well, but determined the molten salt reactor was the best technology. The biggest issues in the commercial industry were economics and nuclear waste.
3 - Molten Salt Reactor Design
Bret Kugelmass: How much impact does the anti-nuclear community have on the public, which has limited knowledge about nuclear?
Ed Pheil: Anti-nuclear communities typically drag out the reactor licensing and construction process. One of the reasons the AP-1000 died is because the process was drug out so long and the financing for construction was so large that the interest doubled the cost of the plant. A similar strategy is used in gas pipelines. One problem the nuclear industry has is that they don’t communicate their position very well. Publicity and media can be used to the benefit of the industry to explain to the public why the people that have the soapbox, the anti-nuclear community, are not correct. The number one priority of Elysium is to reduce cost, but other priorities include solve some of the political issues, spent fuel, reprocessing, and safety issues. Elysium’s design is a molten chloride salt fast reactor. Most salt reactors are starting up with high assay, low enriched uranium between 5-20% enrichment. Since there is currently none of that available in the U.S., Elysium went for plutonium and spent fuel because the U.S. has 61 tons of what the government considers weapons-grade waste plutonium. Fission products aren’t removed intentionally in the Elysium design, even though some fall out naturally, such as gases that bubble off when melted. The spent fuel will be split long ways, chopped into pieces, and poured into a molten salt vat. One of the components of the molten salt will react and grab all the oxides and precipitate out.
4 - Elysium’s Fuel Design
Bret Kugelmass: Is new plutonium added to the spent fuel dissolved in the molten salt to up the enrichment?
Ed Pheil: For the reactor startup fuel, raw plutonium, which is in excess in the U.S., is added to the molten salt after spent fuel is dissolved in it. After startup, plutonium doesn’t need to be added. It is not considered reprocessing because there are no separations of the fuel. The fission products don’t absorb all the neutrons since it is not a fast reactor. The Elysium reactor is a giant cylinder filled up with molten salt, without rods or moderators. A thermal reactor has a moderator in the core. The neutrons are born around 2 meV and are slowed down by the moderator to thermal where the cross-section is much higher and less fuel is needed to go critical. A fast reactor doesn’t slow the neutrons down as much and the reactor operates around 1 meV. Plutonium releases 3 neutrons per fission, compared to uranium-235 which releases 2.4 neutrons per fission. Plutonium-239 is fissile and will fission when introduced to a neutron. Plutonium-240 is fertile and has a high spontaneous fission probability, which inhibits the use of reactor-grade plutonium in weapons. Uranium fuel is not very expensive and there is excess supply. Nuclear fuel is one-third of the cost of operations. However, Elysium is using weapons-grade waste and storage is expensive.
5 - Strategy for Extending Reactor Lifetimes
Bret Kugelmass: What percentage of the fuel is used at the end of its lifetime?
Ed Pheil: The lifetime of the fuel is infinite. The liquid salt repairs itself five to six orders of magnitude faster than the radiation damages it. Every 40-100 years, fission products need to be removed and put back in the reactor. At the end of life of the reactor and there is too much radiation damage, the fuel could be disposed or put in another version of the reactor. The Elysium reactor is designed to continuously replace all the components. If the fuel is continued to be used, 99% of the fuel can be used. In a fast reactor, the cross-section is a lot lower and all the neutrons stay at high energy, so the flux must stay higher to keep the same fission rate. The neutrons go into the materials, hit the iron or nickel atoms, and knock them out of their lattice structure. Nickel creates helium and at high temperatures, helium migrates to the grain boundaries and allows cracking if there is build-up. Over a long period of time, materials creep. The reactor pressure vessel is expected to last 40 years. Leaving fuel in for 40-100 years assumes iso-breeding. Enough fuel is made to replace the fuel being consumed plus fission products. The Elysium reactor could also operate with high assay, low enriched uranium, but the cost of enrichment is very high.
6 - Commercialization Process for Elysium Reactor
Bret Kugelmass: What’s the total power output of the plant you envision?
Ed Pheil: The maximum megawatts electric for Elysium’s system is primarily set by grid size. A 10 MW thermal initial test reactors is planned for startup. A bill was just introduced in Congress that makes low assay, high enriched uranium available for advanced reactors, but Pheil also needs access to plutonium. The Nuclear Regulatory Commission (NRC) has said they can review a license for a molten salt reactor in one year. The reactor vessel has the same volume whether it is at 10 MW thermal or 3,000 MW thermal because the fissile content to be critical is the same. The other half of the fuel volume changes with power. Savannah River or Yucca Mountain would be potential sites, but Yucca Mountain won’t be ready in time. Savannah River has the weapons-grade plutonium and spent fuel can be shipped in. The heat exchanger and pump will probably be built for 500 MW and operated at 10 MW for the initial license. As soon as it is operating at 10 MW, Ed Pheil and Elysium would apply for a uprate license to something like 125 MW thermal. The Elysium molten salt reactor can also operate as a fast test reactor and an isotope production reactor. The core doesn’t need to be designed around the test cells; the test cells can be put anywhere in the core and more fissile is added to compensate for the absorptions. Elysium’s goal is to drive down cost with low pressure, low cost vessels. If the system gets a leak, it chemically bonds and freezes and gases are constantly released. The system operates at very high temperature and can operate at high or low efficiency. Instead of a four-and-a-half year fuel cycle, the fuel cycle is only limited by reactor vessel component replacement.

1) Jarret’s background in journalism and his personal path in the nuclear industry
2) How writing about nuclear has evolved over the last few decades
3) A deep dive into media tactics in nuclear industry communications
4) IAEA and international nuclear audience communication needs
1 - Path to the Nuclear Energy Institute
Bret Kugelmass: Tell us about your background.
Jarret Adams: After college, Jarret Adams went to journalism school and started off his career as a business journalist, covering sectors like aerospace, defense, chemicals, and power. He wanted to make a living through writing and thought business journal provided a way to dig in deeper than general news and work internationally. Adams wrote about topics such as mergers, acquisitions, and new products. He would do background research, conduct interviews, maybe talk to an investment analyst, and use all the information to put a story together. Different industries operate similarly, but each industry has its own unique culture. There is an art to writing and there are tools that allow it to seem more seamless and do it more quickly. Adams spent ten years as a business journalist as the media landscape changed. Daily newspapers were disappearing and turning to online media and social media was introduced. While Adams was working for a tech business magazine in London in 2002, he got laid off and followed an ad to the Nuclear Energy Institute (NEI). The original nuclear renaissance was happening and in 2005 the Energy Policy Act promoted some key policy measures for nuclear. The loan guarantee and production tax credits helped launch Vogtle and Summer.
2 - Communications in the Nuclear Industry
Bret Kugelmass: What were some of your first jobs at the Nuclear Energy Institute (NEI)?
Jarret Adams: Jarret Adams’ early roles at the Nuclear Energy Institute (NEI) were focused on writing. He wrote NEI’s newsletter, “The Nuclear Energy Overview”, the longer form “Nuclear Policy Review”, and various speeches. Adams learned all about the nuclear industry and found it really fascinating. The audience for these NEI publications were the industry and NEI members, who were interested in updates, analyses, and the position of the nuclear industry. NEI has recently opened up the information from members-only to available to everyone. Last year, Areva split into Framatome and Orano. Framatome does fuel fabrication, reactor construction and services. Orano does mining, enrichment, and decommissioning. Jarret Adams worked for Areva in communications and media relations in the U.S. and Canada, where Areva’s largest business activity is uranium mining. Areva had some renewables at the time, but was focused on a pro-nuclear message. Even renewable energy advocates recognize that going for a hundred percent renewables may not be the smartest way to go. It is still good to have a portfolio of energy technologies. Nuclear energy is carbon free, can be used 24/7, is reliable and energy dense, and can do a tremendous amount of energy in a small area. There are still other exigencies which may need a different energy source. Adams has been exposed to all aspects of the entire fuel cycle and the entire nuclear industry through his roles in communications.
3 - Challenges and Benefits of Nuclear Advocacy
Bret Kugelmass: Does the variety of units used in nuclear energy provide unique communication challenges?
Jarret Adams: The nuclear industry uses different units for measurements at different phases of the nuclear cycle. The job of communications roles is to try to explain these measurements to the general public and to convert those things into meaningful information for people. Jarret Adams left Areva in 2014 and spent some time in the UAE. During his time away from nuclear, Adams had an epiphany that, if nuclear had placed a higher priority on its communications and public relations strategies, the industry would be in a different place. Nuclear needs to change some of its messengers by bringing younger, more diverse, and more fresh perspectives. Stories can be told through different media, which is a tactical decision, but the communications need to a part of the core function. Having a consistent brand message across different audiences is important, and your company’s brand should be recognizable. Building the Vogtle plant is tremendously important for America’s future, not just because America needs to demonstrate that they can build a new nuclear plant, but the U.S. needs to retain its ability to do big nuclear projects. The communities around nuclear facilities are extremely supportive and understand the technology. Jobs and economic impact is a core message for stories in the community, but the challenge is getting the stories about nuclear’s importance to places where there aren’t nuclear plants. Unless a wider array of the public is convinced about nuclear, the industry will not succeed because the public has a greater ability to influence where things go.
4 - International Nuclear Growth
Bret Kugelmass: How do we reconcile an industry that wants to grow with an overall decreasing energy demand?
Jarret Adams: The U.S. has slow economic growth, but there is a lot of potential for growth internationally. New projects, like renewables, are still being built in the U.S. because the market is demanding it over other technologies. Nuclear needs to be in a situation in which it is considered a viable solution. Jarret Adams has done some work with the International Atomic Energy Agency (IAEA) talking to newcomer countries who are interested in doing nuclear energy and providing some of the U.S. experiences. When new nuclear is being built, stakeholder outreach is one of the first things that needs to be done. Full On Communications’ role with the IAEA is counseling their representatives, such as the minister of energy or the utility, so they can go out and explain nuclear through town halls, articles in the local newspapers, or interviews on television. Different communication settings are more comfortable in different cultural situations. Nuclear energy is one of the most important technologies for meeting energy needs while fighting climate change. To meet its potential, people must want the technology and there must be a higher priority placed on doing the marketing, public relations, and communications work to recognize nuclear’s great assets.

1) Seth Grae’s background in law and personal journey in the nuclear industry
2) The origin of LightBridge and its consultative history
3) Challenges that are driving the future focus of LightBridge
4) Next steps for commercialization of LightBridge’s new fuel technology
1 - Path to Nuclear Through Law
Bret Kugelmass: How did you get into the nuclear space?
Seth Grae: While in law school, Seth Grae decided he was going to pro bono represent an actual dissident or refusenik in the Soviet Union as part of a final class paper. Through help from people at the State Department and NGO’s, Grae identified a guy who designed the guidance systems for Soviet cruise missile and nuclear submarines. With the support of a major law firm, he went to Russia and filed a brief on his behalf with other American lawyers. This was the first time Grae met American and Russian nuclear engineers and learn about the field. Since he was fascinated by nuclear, he began to gravitate toward high technology international transfer and nuclear, including export control. Going through that experience early on in Grae’s career was very entrepreneurial. In 1984, a neutronics expert named Alvin Radkowski met with his former professor, deciding to go back to a thorium idea for fuel to help spread nuclear power internationally while reducing the chances of making a nuclear weapon. Radkowski asked Grae if he would help him as a lawyer with the thorium fuels. Grae met a nuclear engineer who had the idea for making a thorium fuel that would work in existing reactors, instead of modifying the reactor to take the fuel idea. This eventually became Lightbridge.
2 - Formation of Lightbridge
Bret Kugelmass: What did you do next with the thorium fuel idea?
Seth Grae: Seth Grae formed Lightbridge initially with Andrew Mushakov, who is currently the executive vice president in charge of nuclear operations at Lightbridge. Develop a new nuclear fuel is a lot less expensive than developing a whole new kind of reactor, but it is still very expensive for a startup company. They had to come up with a way of making tens of millions of dollars. In 2007, they had an idea for a company that should exist in the world that didn’t that could produce a lot of revenue. There was no company on Earth that could guide a country that never had reactors how to get one. The big entities, like the International Atomic Energy Agency (IAEA) couldn’t do it and the big companies like GE and Westinghouse didn’t have a business unit that could do it. Through a very strong advisory board connection, Grae and his team talked about the idea for a nuclear power program with the United Arab Emirates (UAE). The government of UAE and Abu Dhabi hired them to write the nuclear power plan for the UAE. Before the UAE contract, it had been over 30 years since a country that had never had reactors decided to get them and actually built them, which was China. Grae decided to take Lightbridge to NASDAQ to be publicly traded to provide a means for his investors to buy liquid stock in the company.
3 - Proliferation Risks of Nuclear Fuel
Bret Kugelmass: How have you taken an out-of-the-box approach across multiple aspects of Lightbridge?
Seth Grae: Dan Magraw has been on the Lightbridge Board of Directors since the beginning. He gives Lightbridge the only leading environmental policy figure on the board of directors of a nuclear energy company. Lightbridge ensures that they are transparent by being publicly traded. So few purely nuclear companies are publicly traded and publish all their financials. Lightbridge also focuses on transparency by putting a patent on their nuclear fuel design. A group of nuclear scientists and engineers at Virginia Commonwealth University (VCU) wrote a peer-reviewed article showing how, of all the nuclear technologies looked at for nuclear power and nuclear fuels in existing reactors, only one could not be usable by would-be proliferators: Lightbridge. This result would not have been possible if Lightbridge had protected their design with trade secrets. When uranium is enriched to make traditional fuel, one isotope is taken out of uranium that can’t be used to make bombs and making the fuel a higher percentage of the isotope, uranium-235 that can make bombs. Uranium-235 is about 0.7% in mined uranium and fuel is usually enriched up to 4.95%. A bomb can be made with enrichment over 90%. When the reactors are refueling and used fuel is being taken out of the reactor, some of the uranium-238 becomes plutonium-239 which is a weapons-usable isotope. The plutonium can be extracted. To make a bomb from fuel that comes out of a commercial reactor has not been done and would be an expensive and difficult process. The Lightbridge fuel reduces the amount of plutonium-239 by burning it as it’s created to make power and mixing it with other substances that make it unable to make a bomb.
4 - Nuclear Economics and Safety
Bret Kugelmass: At what point did you decide to go down the path of developing a fuel technology?
Seth Grae: Lightbridge thought their idea had great safety benefits. It reached back to things the U.S. government had down decades earlier in what was called delta phase, metal fuels. These ideas were brought back in an advanced way that no one had done before to not have another Fukushima. The meltdown at Fukushima in March 2011 was traumatic from a business standpoint in losing four billion dollar facilities and the way it was portrayed as a disaster where no one got hurt and no one died. Not long after Fukushima, the collapse in nuclear gas prices destroyed nuclear in the merchant plants and deregulated markets. The combination of these two events invigorated Seth Grae and Lightbridge to have technology that could solve these problems for existing reactors and new ones that get built. Jim Malone came to Lightbridge from Exelon, who was the largest buyer of nuclear fuel in the United States. Lightbridge wanted to solve five problems. They wanted to dramatically improve the economics of existing reactors, more than the industry imagined they could. Lightbridge also wanted to dramatically improve the safety of the already safest industry in the United States. Safety could capture the public’s imagination by lowering the fuel temperature by 1000 degrees Celsius. Coping is the time the operators of the reactor have to deal with an accident or off-normal accident and lower temperatures buys the operators more time.
5 - Load Following and Life Extension in Nuclear
Bret Kugelmass: How did you find a way to cool the decay heat over time?
Seth Grae: Dumping heat through metal, which has thermal conductivity, transfers heat faster and better than the current fuel. Lightbridge wanted their fuel to be used anywhere in the world without any worry about anyone using a nuclear weapons program. The current technology is fantastic in that area, but Lightbridge is leaps and bounds better. Reactors are particularly bad at load following; they are good when they are run at a constant rate. Load following is suddenly an important thing because of renewables which only provide power at a certain time of day. The current fuel has uranium pellets stacked up inside a tube. When the power is turned on or power increases, the pellets swell and can even crack the tube. Lightbridge doesn’t have pellets inside a tube. Lightbridge also aims to extend the life of the reactors beyond what they have already run. If the reactors make a lot more money and producing more power with Lightbridge fuel, there is an economic case for keeping the reactor going as opposed to losing money. Physical changes can also help plants run longer. In license renewal, Nuclear Regulatory Commission (NRC) looks at whether the pressure vessel can operate for another 20 years safety. The pressure vessel is the first line of defense if there is an accident, but gets brittle over time and gets stressed every time a reactor is turned off and on again. By having a fuel that lasts a lot longer between refueling, the pressure vessel is depressurized and re-pressurized a lot less. The vessel also goes through irradiation and thermal cycles.
6 - Collaboration Between Lightbridge and Utilities
Bret Kugelmass: What was the design process like for Lightbridge fuel?
Seth Grae: Lightbridge had an initial concept based on what the U.S. had done decades earlier and other countries had started to run with some metal fuel ideas for different applications. The U.S. never stopped developing and using metal fuel technology, but they didn’t transfer it into the commercial sector. Lightbridge has scooped up experts who worked at National Labs, who have the world-leading expertise in metal fuel. New kinds of reactors will take a very long time to deploy, so until that time comes, the current fleet needs to keep operating. With Jim Malone’s help, Seth Grae and Lightbridge wanted to meet the huge problems of the industry after Fukushima and collapsing gas prices. Four buyers - Exelon, Duke, Dominion, and Southern Company - dominate as utilities and nuclear owners and operators and collectively have half the nuclear power plants in the U.S. They have every kind of reactor, fuel supplier, fuel chains, and NRC licensing. With Jim Malone’s help, Lightbridge formed the Nuclear Utility Fuel Advisory Board (NUFAB) which consists of the heads of nuclear fuel of Exelon, Duke, Dominion, and Southern Company. The group gets together and, from a design point of view, looks at how to solve problems and use it in their reactors, without changing anything in the nuclear reactor. The utilities submitted a document to the NRC expressing their interest and asking the NRC to begin to prepare license applications. Fuel manufacturers showed interest because the utilities showed interest. Lightbridge negotiated a fantastic deal with Framatome who brought everything needed to finish the commercial design, license it with the NRC, manufacture it in America, and sell it in domestic and export markets.
7 - Innovation in the Nuclear Space
Bret Kugelmass: Tell me about what you provide to Framatome.
Seth Grae: There has never been a truly differentiated product in nuclear fuel for the existing reactors. The ability to have a differentiated product that’s not a commodity that brings dramatic improvement and benefits is the goal of Lightbridge. The United States Department of Energy (DOE) has been there during the life of the project and is taking steps to lift up American innovation to have America come back in nuclear power, not just domestically, but globally. The DOE started late in the Obama administration looking into something that became funding grants to help companies. The U.S. government has done something similar in 2006 for launching rockets and satellites and started funding companies in 2008. At the time, the Russians were dominating the multi-billion dollar global industry. Ten years later, Russia is out of the commercial satellite launch industry internationally and SpaceX and others, who have better technology that got funded by the American government, crushed them. In nuclear, the government is not the customer. NuScale is designing a fantastic advanced reactor that runs with light water reactor fuel and is the world leader on small modular reactors (SMR). The DOE is disproportionately highly funding NuScale because it funds the whole advanced reactor industry in the U.S. and can help NuScale get a first customer at Idaho National Laboratory with UAMPS (Utah Associated Municipal Power Supply). Lightbridge started with the customer.
8 - Lightbridge Commercialization Process
Bret Kugelmass: What are the next steps toward final commercialization of Lightbridge’s product?
Seth Grae: In the coming months, Lightbridge is going to see how the government funding applications go and announce their first major contract with a major utility to put skin in the game. The fuel is going into the lead test rod assembly program in 2021 and is announcing, with the DOE and the National Labs, a research reactor test program that will start in 2020 that will feed samples going into the TREAT reactor. Lightbridge is going to make four meter-long rods that will be put together into one commercial-length rod that will have different zones up and down the rod. It will be put inside an actual commercial reactor in the U.S. to see how the four different areas do in parallel with the 2020 tests in the research reactors. This combination of data will give comfort to the utilities that it provides a benefit to the reactors and to the Nuclear Regulatory Commission (NRC) that they have the data they need to license it. The fuel will also go into the fuel pool, travel into the core, provide power, and be irradiated by the assemblies around it. The fuel will be taken back out to the spent fuel pool providing commercial experience and approvals. A whole assembly will go in 2023 or 2024 as part of the lead test assembly program, per a letter of intent from a major utility. In 2027 or 2028, Seth Grae anticipates full commercialization. Fossil fuels causes air pollution and climate change, but people give it a market because it is easy to extract. An independent study by Siemens showed that Lightbridge is going to destroy fossil fuels in the marketplace though base load power. The cheapest way to make massive base load power it to switch a reactor to Lightbridge fuel. It will be way cheaper than coal, natural gas, and renewables with the best non-proliferation on the planet. Nuclear at its worst is safer than coal at its worst. The United Arab Emirates (UAE) will be the next country to start up nuclear reactors in the world and is meeting and exceeding the highest standard in the world. Without growth in nuclear power, the world won’t meet climate, air pollution, or energy goals. Lightbridge can provide this growth without building the reactors, but instead getting more power out of the existing ones and justifying the case to build new reactors.
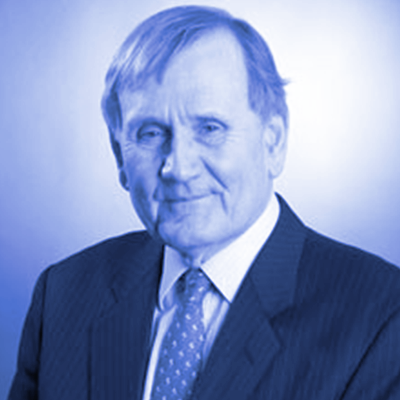
1) Thomas’ personal journey from his position as United States House Committee on Banking and Currency to Ambassador of the US Arms Control and Disarmament Agency
2) How nuclear energy actually played a vital role in halting the spread of nuclear weaponry
3) Thomas’ work advocating nuclear and his book Seeing the Light
4) Nuclear’s many environmental benefits including the prevention of climate change
1 - International Law & Politics
Bret Kugelmass: How did you get started in your professional career?
Thomas Graham: Thomas Graham is from Louisville, Kentucky and studied at the Woodrow Wilson School at Princeton University in the international section. Graham went to Paris for a year to study at the Institute of Political Science and, when he came back, was accepted into Harvard Law School. Since he wasn’t in a continuous educational program, Graham was drafted into the Vietnam War. He spent two years in the U.S. Army, getting out in 1958. Graham had to apply to Harvard Law School for a third time and was finally able to attend. After his time at Harvard, Graham clerked for the chief judge of the DC circuit, worked for the Treasury, counseled the House banking committee, did private practice in Louisville, and joined a large international banking law firm in New York. The 1968 election came along and Graham went to the Democratic Convention with the Kentucky delegation as a hanger-on. Graham decided he could no longer support the Democratic party and wrote to his cousin, Nixon’s Defense Secretary, in search of a job in Nixon’s administration. He got a job in the next campaign and worked for a year in the Air Force General Counsel’s office working on radar systems in Northern Canada. Graham heard about an agency within the State Department whose primary responsibility was negotiating nuclear weapon agreements with the Soviet Union. On his first day of work, Graham was briefed on the status of the SALT negotiations and was fascinated.
2 - SALT Negotiations
Bret Kugelmass: Tell us about some of the work you were involved in at the State Department.
Thomas Graham: Thomas Graham was very involved with the first round of SALT, creating political support for the anti-ballistic missile treaty and the agreement on strategic offensive arms. He was the counsel to the delegation that wrote the treaty. He did the first draft of the SALT II treaty with the delegation in Geneva. The draft was sent to the National Security Council and Graham followed to be available for discussion on the treaty. Graham found working on the negotiations very interesting since there were so many different issues that came up. The anti-ballistic missile (ABM) treaty was negotiated during SALT I and became a big issue during the Reagan administration. Its provisions prevented what was called “Star Wars”, or the Strategic Defense Initiative (SDI) from being deployed. Things got tough as people got in legal trouble for obstruction of justice, planting things in offices, and leaking classified information to columnists. At one point, Graham was reported to the FBI by the right wing guys, leading Graham to get a lawyer regarding an interview he gave to The New York Times. He was accused of leaking classified information, since it was published in the article, but Graham was not the source and even had a witness during his interview.
3 - Conventional Armed Forces in Europe Treaty
Bret Kugelmass: Is it easy to get in trouble in the political realm?
Thomas Graham: After Thomas Graham’s second time around with the FBI, Graham was warned by one of the agents that some guys were out to get him. He was also the target of a Senator who said unfriendly things about Graham on the Senate floor, but Graham understood it was part of the game. In politics, if you play by the rules and stick close to them, everything will be okay in the end. The extension of the non-proliferation treaty was an interesting exercise in which Graham went around the world rounding up votes. He had to have many reasons why these countries should support the U.S. position; his strategy was to go straight to the minister in the capital instead of dealing only with their representatives in New York. One other negotiation Graham participated in was the Conventional Armed Forces in Europe Treaty. This treaty and negotiation essentially ended the Cold War at the working middle level. During this negotiation, they started out with 16 NATO countries and seven Warsaw Pact countries, 23 total countries. Five countries changed their name and one, East Germany, disappeared. The Soviet Union fell apart, Czechoslovakia split apart, and the negotiation ended up with 30 countries. The number of tanks and other conventional weapons had to be brought into balance.
4 - Extension of the Non-Proliferation Treaty
Bret Kugelmass: What could have happened in the Cold War?
Thomas Graham: There could have been a better settlement with Russia at the end of the Cold War. The U.S. was tough on Germany after World War I, but easy on Germany and Japan after World War II. Russia should have been treated how everyone was treated after World War II and the U.S. could have ended up with a democratic ally. The extension of the non-proliferation treaty (NPT) was a very interesting and very rewarding experience. It was a demonstration that approaching people the right way by trying to understand their point of view made the impossible possible. Thomas Graham prioritized building relationships with other countries, including the Mexican and Russian delegations. One of the big issues during the conference was some kind of restraint in the Middle East. The night before the final agreements, Graham tried to work something out with Egypt and Syria. The final agreed upon language was that there should be an additional resolution that there should be a freeze on nuclear weapons and weapons of mass destruction in the Middle East. Depositaries still exist in the non-proliferation treaty because it was negotiated during the Cold War.
5 - Peaceful Uses of Nuclear Energy
Bret Kugelmass: How did we deal with the threat of potentially unemployed nuclear scientists?
Thomas Graham: Thomas Graham’s company, then called Thorium Power and now called Lightbridge, was founded in the early 1990’s when nuclear was a threat. They were developing a fuel based on thorium, which had a lot of positive attributes in terms of the nuclear fuel cycle. One variant is the thorium variant and the other is all uranium. Graham first joined the board as he retired from the government around 1997, near the end of the Department of Energy (DOE) support for the company. The DOE had provided capital to the company for five or six years since the company hired many Russian nuclear scientists, as many as 500 at one time, as consultants during fuel development. The fuel was developed at Kurchatov Laboratory in Moscow and there were very close relationships with the management of the laboratory and many senior Russian scientists for many years. Graham had always been interested in nuclear energy because the nuclear non-proliferation treaty covers peaceful uses of nuclear as well, not just weapons. The peaceful uses of nuclear energy were an arrant right of all nuclear non-proliferation treaty parties. Nuclear medicine was also an influential factor for countries that may not need nuclear power, such as Belize. Some countries believe that mastering the nuclear fuel cycle and utilizing it for energy is one of the highest scientific achievements that humanity has ever made, and if they can do it, they are just as good as anybody else. Nuclear power was not being treated properly by the media and there was hysteria about the danger of nuclear reactors. Statistically, nuclear reactors are the safest form of energy production that exists, based on damage, injuries, and death. Nobody died from anything nuclear at Fukushima or Three Mile Island and the UN estimates that 62 people died as a result of Chernobyl. However, 90,000 people died in coal mines from 1910 to 1940 or 1950.
6 - A Nuclear Solution to Climate Change
Bret Kugelmass: Is radiation treated much more seriously than other things that hurt people and does that scare people?
Thomas Graham: People have nuclear fear which goes back to the explosion at Hiroshima. Everyone gets radiation every day and human bodies have learned to regard a certain level of radiation as positive. Even most elevated levels are not harmful, including exposure during an airplane flight. Radiation of CT scans and x-rays are also common. The attitude towards radiation is way overdone. It must be watched and treated carefully, but no more so than lots of chemicals. Humanity is under the most dangerous threat it has ever faced: climate change. It is an existential threat of enormous proportion and, even if the U.S. had stayed in the Paris agreement, it would not have been possible to keep the level at two degrees Celsius over the heat level of the Earth. The only solution that we know of today is the massive use of nuclear power. Renewables and reforestation need to happen too, but nuclear has got to carry most of the load unless people want to go back to the Stone Age.
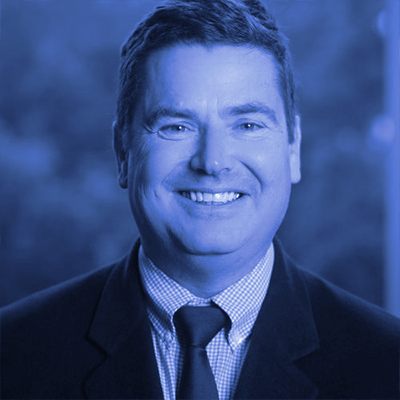
1) David’s work as Director for the U.S. Department of Energy innovation hub, Consortium for Advanced
2) PWR and BWR reactors
3) Independent analysis of reactor core design and fuel optimization
4) The Future of CASTLE and its contributions to the nuclear industry
1 - Computational Reactor Physics
Bret Kugelmass: Where did you grow up?
Dave Kropaczek: Dave Kropaczek grew up in New Jersey and studied engineering science at Newark College of Engineering, now called the New Jersey Institute of Technology. Within engineering science, Kropaczek minored in nuclear engineering after a visit to the Tokamak reactor at Princeton while in high school. He went on to receive his Master’s and PhD at North Carolina State University. During his industry-sponsored Master’s degree, Kropaczek spent nine months in school and nine months at a sponsor site, Westinghouse in Pittsburgh. Kropaczek went back to Westinghouse after his PhD and was exposed to two-thirds of the reactor fleets in terms of solving engineering problems. Everything has a process, but there is still a lot of creativity allowed. The fuel within the core is reconfigured every cycle, as the highest exposed or highest burnt fuel is replaced with new fuel and it is all shuffled and reconfigured to get the maximum energy out. The utility is the end customer and has to produce a certain amount of energy over time and multiple cycles and outages are planned years in advance. Westinghouse is also a nuclear fuel vendor. As Kropaczek was in his Master’s level nuclear classes, he became more interested in nuclear methods, leading him to become a computational reactor physicist.
2 - Boiling Water Reactor Core Design
Bret Kugelmass: How does temperature affect cross-sections?
Dave Kropaczek: Everything has a temperature that impacts the number densities of water. If the number density changes, the moderation or slowing down of neutrons to thermal energy takes place, which is a feedback effect. There is negative feedback when water is boiling and the reactor is in accident mode. Light water reactors are designed to have a negative reactivity. The reactor is designed for a thermal spectrum, so if the neutrons can’t be slowed down, the uranium is kept below a certain enrichment limit. The decay heat must go somewhere, and since the fuel rod has no water to cool it, it will eventually fail and fuel will melt. In a loss of cooling accident (LOCA), active systems must come on and get water into the reactor. The coolable geometry must be maintained as part of a transient analysis. Dave Kropaczek also worked for General Electric in global nuclear fuels, a joint venture of General Electric, Toshiba, and Hitachi. Boiling water reactors have different issues of thermohydraulic stability. Kropaczek worked on optimization codes to design assemblies in reactor cores to get the best economic value. Boron is used as a dilution process, which is eliminated in boiling water reactors. The control mechanism is the void itself and the void feedback is a great control because it decreases a moderation of neutrons which tends to slow down the reactions.
3 - Studsvik Scandpower
Bret Kugelmass: Was Global Nuclear Fuels looking at supplying fuels to boiling water reactors that don’t exist yet?
Dave Kropaczek: The ABWR (American Boiling Water Reactor) and ESBWR (Economic Simplified Boiling Water Reactor) are both General Electric (GE) developed power plants, as GE was at the forefront of boiling water technology. Fuel vendors have a set of nuclear analysis codes. Dave Kropaczek went to work at Studsvik Scandpower as a co-developer, a company that evolved from the National Laboratory in Sweden. Technology was developed at Studsvik that was brought to the U.S. and commercialized. The commercial model for Studsvik Scandpower software was that it was independent of the fuel vendors, giving the utilities an independent analysis capability. Different fuel products from different fuel vendors can be analyzed by things like flow behavior in a bundle or fuel rod diameters. The configuration of fuel bundles determines power output and what the control will look like. The applications of Scandpower codes include licensing, design, or other areas across the spectrum, but there must be permission to deliver products outside the U.S. Kropaczek came in to Scandpower in a technical role, but jumped into a strategic leadership role also responsible for profit and understanding international sales. The U.S. base for Studsvik was in Wilmington, North Carolina.
4 - The CASL Energy Innovation Hub
Bret Kugelmass: What prompted the teaching role you took at North Carolina State University?
Dave Kropaczek: Dave Kropaczek learned a lot and enjoyed the CEO role, but wanted to be more in the technical realm of things. He took a teaching role at North Carolina State University and was also the chief scientist of CASL (Consortium for Advanced Simulation of Light Water Reactors). Kropaczek taught one class a year and also advised senior design classes and individual graduate projects. CASL was formed in 2010 and was the first energy innovation hub focused on nuclear energy. It was initially a five year program that looked at whether advanced modeling simulation could be used to attack problems of interest in the nuclear industry. The U.S. nuclear fleet is very safe and has high capacity factors. CASL was a partnership between the National Labs, industry partners, and academia. The challenge problems for CASL have remained fairly constant, with the number one challenge problem still being crud in nuclear reactors. Crud is the deposits of nickel and iron compounds on the surface of the fuel rod in the presence of sub-cooled oil. It is related to the chemistry of the reactor coolant and the corrosion of the piping systems. In pressurized water reactors (PWR’s), the soluble boron in the coolant come out of the solution and become a solid in the crud.
5 - Local and Global Impacts of Crud
Bret Kugelmass: What happens when crud collects the boron?
Dave Kropaczek: When boron collects on crud on the fuel rods, the operating power of the nuclear power plant starts shifting down to the bottom of the core. If there is a significant event in which a temporary shutdown needs to happen, the boron comes out of the crud layer back into the solution causing it to disappear. It can never be seen in the reactor, but the impacts can be observed during operation. When the reactor is started back up, the boron is gone and the power is no longer suppressed, causing the power to shoot to the top of the core and the axial offset swing flips, called crud-induced power shift. Crud-induced localized corrosion can impact the heat transfer around the fuel rod, degrading the heat transfer where the cladding can heat up and fail. Crud has local and global effects in the reactor. To flatten the power in the core, more burnt fuel has to be removed and more fresh fuel has to be installed, causing the fuel to not get to the high level of burn up as it needs to for economic reasons. Pellet clad interaction is another challenge issue studied at CASL. The fuel pellet can expand rapidly inside the cladding and the clad can creep down onto the fuel pellet. If the expansion is rapid, the clad can be stressed and fail. Water is very hard to model in terms of fundamental physics, so a departure from nuclear boiling challenge focuses on computational fluid dynamics of the boiling of water on the surface of fuel rods and potential dry outs.
6 - Departure of Nucleate Boiling
Bret Kugelmass: How do you see the departure of nucleate boiling (DNB) inside a reactor?
Dave Kropaczek: Partial dry out of a fuel rod, departure of nucleate boiling (DNB) looks a different color and may not have failed, but instead been in a poorly degraded heat transfer state in which it starts to morph into something that’s not supposed to be there. Large scale facilities have heater rods that simulate the departure of nucleate boiling. The products are tested in the facilities and correlations are developed for critical power or DNB, based on the functions of mass flux or various thermohydraulic parameters. The correlation is developed for the product and applied to the code. In the DNB challenge problem, testing could be replaced or supplemented with a fundamental, first principles method. CASL is working closely with NuScale’s small modular reactor (SMR) design, looking specifically at modeling crud in the reactor to potentially address issues with the natural circulation in the design.
7 - Mission of CASL
Bret Kugelmass: What do you want to see happen at CASL before and after the end of its second extension?
Dave Kropaczek: Dave Kropaczek’s primary goal at CASL is to complete the challenge problems and provide the solutions to industry. To deploy technology, there are multiple requirements on software such as insurance and usability. CASL is now looking at deployment in a commercial setting and how advanced modeling simulation tools fit in the process. The team is working with industry to find the high value, high impact problems that CASL can aid in solving. CASL’s mission has moved from fundamental research and development early on to development and deployment now, with a focus on getting codes out to industry. CASL has a sister program for advanced reactors called NEAMS (Nuclear Energy Advanced Modeling & Simulation). The vision is to have a new program that looks at whether advanced modeling and simulation could be used in areas that haven’t been imagined yet, combined with data analytics. There is a plan to get a post-CASL user community into a self-sustaining entity. Kropaczek’s focus is delivering on what was promised at CASL. Plants are well-maintained now and there is tremendous economic pressure. A new nuclear renaissance is needed. Small modular reactors (SMR’s) lower the capital cost, allowing competition and modularity. The safety systems in the new AP-1000 are way beyond the existing fleet, which are already completely safe. If there were a large demand for SMR’s, the cost would come down.
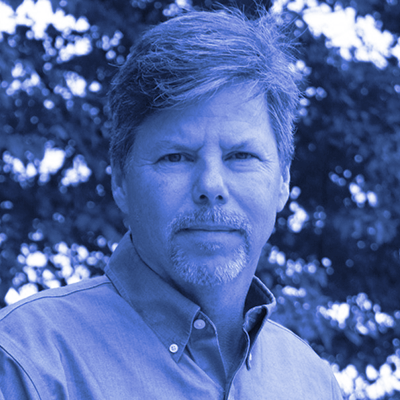
1) Lou’s background in fusion and personal journey in the nuclear space
2) Challenges in nuclear fission
3) The resurgence of interest in molten salt reactors
4) The Advanced Reactor Technology program within the U.S. Department of Energy
1 - Pellet Injection into Plasma
Bret Kugelmass: What set you on your current path?
Lou Qualls: Lou Qualls grew up in West Tennessee and went to school at the University of Tennessee at Knoxville, receiving his PhD in nuclear engineering in the fusion area. He developed fueling technology for plasmas. Hydrogen plasma has deuterium and tritium in it. In order to get the fueling into the center of the plasma, it needs to be frozen into a little ball and shot at high velocity so it melts on the way in, which is called a pellet injection system. In his PhD work, Qualls worked on a stellarator the Advanced Toroidal Facility (ATF), a research project at Oak Ridge National Lab (ORNL) at the Y-12 site. A stellarator is a fusion device that has current carried in the coils so it can run in steady state. The alternate type is called a tokamak, like ITER, and is run in an inherently pulsed mode. The tokamak makes a better plasma, giving it the most promise to make a fusion reactor compared to the stellarator. When Japan built tokamaks, they found the physics changed when it was scaled up. Qualls hired into Oak Ridge in the pellet injection group; his job is to shoot pellets into plasma. He worked on the TFTR (Tritium Fusion Test Reactor) at Princeton University as a post-doc for 9 months. Instead of using the tritium pellet injector, they used neutral beam injection. This particle beam of protons goes straight through the plasma. Beams heat the plasma, but pellets cool the plasma. Neutral beams can heat the plasma and also drive the current, which the tokamak needs. Qualls also worked on the Tore Supra overseas in Cadarache. Upon his return to Oak Ridge and settling down in his family life, Qualls switched from the fusion world into the fission world.
2 - High Flux Isotope Reactor
Bret Kugelmass: How did you make your initial connections in the fission world?
Lou Qualls: Lou Qualls eventually switched from the fusion world into the fission world through his network of professional connections. Someone recommended Qualls for a position in the fission division and he accepted an initial job related to building radiation experiments that would go into the HFIR at Oak Ridge National Lab (ORNL). The goal was to test material specimens at various temperatures that would be relevant for the fusion energy program. Qualls worked at the High Flux Isotope Reactor (HFIR), which was first built in 1965. It was designed to produce medical isotopes and heavier than uranium elements. During design, someone had the idea to put beam ports in to provide the capability for neutron beam experiments later on. The shield has a hole which allows neutrons to pass. Materials are placed on a target to undergo experiments with well-aligned neutrons. HFIR is now mostly a science experiment that also makes isotopes and does irradiation experiments. This provides the ability to meet multiple sponsors’ needs.
3 - Space Power & Molten Salt
Bret Kugelmass: How did you get in charge of molten salt reactors?
Lou Qualls: Lou Qualls was doing irradiation while the space program was up and running. The idea for Project Prometheus was to take a nuclear reactor, create electricity, and do electric propulsion for vehicles that would go to the moons of Jupter and get into the gravity weld. Building a vehicle that could orbit for a while, get out, and go to the next moon required a nuclear reactor. When the project and space power program collapsed, but Qualls still thought it was an exciting initiative to work on. The fission surface power initiative was for developing power systems that could be used on the Moon and on Mars. Thermal propulsion is another form of propulsion in nuclear propulsion. Qualls was asked to be the space power program manager at Oak Ridge National Lab (ORNL), which he accepted. ORNL engaged with other national labs and NASA research centers to collaborate across the nation. Around 2010, there was a resurgence in interest for molten salt reactors. In the 1960’s, ORNL hit upon the molten salt reactor as a good, safe, economic possibility for reactor development. It had a high temperature coolant, a low pressure system, and was easy to operate. The molten salt reactor experiment was built to demonstrate the viability of the concept. Molten salt technology was a possible commercial technology, but was competing with other more mature technologies.
4 - Comeback of Molten Salt Technology
Bret Kugelmass: Why did ORNL start looking at molten salt reactors again in 2010?
Lou Qualls: Back in the 1960’s, Oak Ridge National Lab (ORNL) built a molten salt reactor than ran very reliably for a number of years. The technology was demonstrated, but many technical challenges remained and interest and funding shifted to light water reactors. At the same time, the need for breeding fuel lessened. A molten salt reactor is any reactor that uses molten salt to a significant degree in the primary system. A solid-fueled salt-cooled concept is like a traditional reactor except the coolant has been replaced by a low pressure, high temperature coolant that doesn’t require a thick-walled vessel for a high pressure system. The higher temperature gets a higher conversion efficiency in making electricity. Another version of molten salt reactor takes the fuel and dissolves it as a salt into the coolant which flows around the system. There is not a lot of excess reactivity in the system and reduces the possibility of a reactivity event. The poison effect from fission can be minimized. The salts studied back in the day are the same salts being looked at now. Fluoride or chloride salts can be combined with lithium, beryllium, or uranium to make a salt. Chloride-based salts are better suited for fast, high energy neutron spectrums. Lou Qualls is the National Technical Director for a Department of Energy (DOE) advanced reactor technology program, specializing in molten salt reactors. His job is to understand what molten salt developers are doing and to perform research within the DOE system that helps them be successful. Salt material interaction is basic science that can be done to understand what drives corrosion and develop ways to detect and mitigate it. The chemistry of the salt changes over time and the performance impacts of reactor behavior must be understood.
5 - Fission Products and Salt
Bret Kugelmass: Do fission products interact with the salt in molten salt reactors to form new compounds?
Lou Qualls: Fission products are put into the salt as the as the reactor runs and they are tracked over time and grouped into classes. Noble gases come out of the system because they don’t react with anything and a system in the reactor lets them escape. Some fission products react with the salt, the fluoride or chloride, and can become soluble in the system. Soluble products have an impact on reactor behavior, but as more soluble fission products are in the salt, there is less room in the salt for uranium. A solid can be formed within the liquid salt and can nucleate and begin to grow. This same particle could end up in a filter somewhere, leaving the salt but still in the system. Other things can stick to the wall, such as noble metals that don’t react or create salt solutions. This may disrupt the flow, impact the dose in the area, or put a heat load on the component that is serious enough to worry about getting over temperature if not properly cooled.
6 - Design of Molten Salt Reactors
Bret Kugelmass: What are the impacts of a hot spot in a molten salt reactor?
Lou Qualls: Hot spots could create a problem with a specific component. If maintenance has to be done on that component, there is no longer an easy way to access it to do deposits stuck on the wall. Monitoring and maintenance of a molten salt reactor is going to have challenges because of the distributed dose that is around the plant, not just in the fuel in the core underwater. Molten salt reactors don’t have a water pool above them so there must be different ways to shield and access. Camera systems that are tolerant to high doses of radiation and temperature must fit into the primary system. Components can be designed to last the lifetime of the plant or they can be designed to be replaced if they were not going to last the lifetime of the plant. This must be a thoughtful upfront design decision. The normal nuclear business thinks of everything as fixed and permanent, but molten salt reactors are more like a steam plant in which it is rebuilt over the span of its lifetime. The logistics of getting into the molten salt reactor business are challenges. Getting salts fabricated, transported, irradiated must be thought about. Supply chain on the front end must be created to make pumps and heat exchanges for molten salt reactors and have different design rules because of higher temperatures. This information must be shared with the NRC (Nuclear Regulatory Commission) and developers to get it into the design real-time. The nuclear business is in a difficult spot right now and needs a change. Evolutionary change is not always healthy; a revolutionary change is needed and molten salt can bring that to nuclear.
1 - Pellet Injection into Plasma
Bret Kugelmass: What set you on your current path?
Lou Qualls: Lou Qualls grew up in West Tennessee and went to school at the University of Tennessee at Knoxville, receiving his PhD in nuclear engineering in the fusion area. He developed fueling technology for plasmas. Hydrogen plasma has deuterium and tritium in it. In order to get the fueling into the center of the plasma, it needs to be frozen into a little ball and shot at high velocity so it melts on the way in, which is called a pellet injection system. In his PhD work, Qualls worked on a stellarator the Advanced Toroidal Facility (ATF), a research project at Oak Ridge National Lab (ORNL) at the Y-12 site. A stellarator is a fusion device that has current carried in the coils so it can run in steady state. The alternate type is called a tokamak, like ITER, and is run in an inherently pulsed mode. The tokamak makes a better plasma, giving it the most promise to make a fusion reactor compared to the stellarator. When Japan built tokamaks, they found the physics changed when it was scaled up. Qualls hired into Oak Ridge in the pellet injection group; his job is to shoot pellets into plasma. He worked on the TFTR (Tritium Fusion Test Reactor) at Princeton University as a post-doc for 9 months. Instead of using the tritium pellet injector, they used neutral beam injection. This particle beam of protons goes straight through the plasma. Beams heat the plasma, but pellets cool the plasma. Neutral beams can heat the plasma and also drive the current, which the tokamak needs. Qualls also worked on the Tore Supra overseas in Cadarache. Upon his return to Oak Ridge and settling down in his family life, Qualls switched from the fusion world into the fission world.
2 - High Flux Isotope Reactor
Bret Kugelmass: How did you make your initial connections in the fission world?
Lou Qualls: Lou Qualls eventually switched from the fusion world into the fission world through his network of professional connections. Someone recommended Qualls for a position in the fission division and he accepted an initial job related to building radiation experiments that would go into the HFIR at Oak Ridge National Lab (ORNL). The goal was to test material specimens at various temperatures that would be relevant for the fusion energy program. Qualls worked at the High Flux Isotope Reactor (HFIR), which was first built in 1965. It was designed to produce medical isotopes and heavier than uranium elements. During design, someone had the idea to put beam ports in to provide the capability for neutron beam experiments later on. The shield has a hole which allows neutrons to pass. Materials are placed on a target to undergo experiments with well-aligned neutrons. HFIR is now mostly a science experiment that also makes isotopes and does irradiation experiments. This provides the ability to meet multiple sponsors’ needs.
3 - Space Power & Molten Salt
Bret Kugelmass: How did you get in charge of molten salt reactors?
Lou Qualls: Lou Qualls was doing irradiation while the space program was up and running. The idea for Project Prometheus was to take a nuclear reactor, create electricity, and do electric propulsion for vehicles that would go to the moons of Jupter and get into the gravity weld. Building a vehicle that could orbit for a while, get out, and go to the next moon required a nuclear reactor. When the project and space power program collapsed, but Qualls still thought it was an exciting initiative to work on. The fission surface power initiative was for developing power systems that could be used on the Moon and on Mars. Thermal propulsion is another form of propulsion in nuclear propulsion. Qualls was asked to be the space power program manager at Oak Ridge National Lab (ORNL), which he accepted. ORNL engaged with other national labs and NASA research centers to collaborate across the nation. Around 2010, there was a resurgence in interest for molten salt reactors. In the 1960’s, ORNL hit upon the molten salt reactor as a good, safe, economic possibility for reactor development. It had a high temperature coolant, a low pressure system, and was easy to operate. The molten salt reactor experiment was built to demonstrate the viability of the concept. Molten salt technology was a possible commercial technology, but was competing with other more mature technologies.
4 - Comeback of Molten Salt Technology
Bret Kugelmass: Why did ORNL start looking at molten salt reactors again in 2010?
Lou Qualls: Back in the 1960’s, Oak Ridge National Lab (ORNL) built a molten salt reactor than ran very reliably for a number of years. The technology was demonstrated, but many technical challenges remained and interest and funding shifted to light water reactors. At the same time, the need for breeding fuel lessened. A molten salt reactor is any reactor that uses molten salt to a significant degree in the primary system. A solid-fueled salt-cooled concept is like a traditional reactor except the coolant has been replaced by a low pressure, high temperature coolant that doesn’t require a thick-walled vessel for a high pressure system. The higher temperature gets a higher conversion efficiency in making electricity. Another version of molten salt reactor takes the fuel and dissolves it as a salt into the coolant which flows around the system. There is not a lot of excess reactivity in the system and reduces the possibility of a reactivity event. The poison effect from fission can be minimized. The salts studied back in the day are the same salts being looked at now. Fluoride or chloride salts can be combined with lithium, beryllium, or uranium to make a salt. Chloride-based salts are better suited for fast, high energy neutron spectrums. Lou Qualls is the National Technical Director for a Department of Energy (DOE) advanced reactor technology program, specializing in molten salt reactors. His job is to understand what molten salt developers are doing and to perform research within the DOE system that helps them be successful. Salt material interaction is basic science that can be done to understand what drives corrosion and develop ways to detect and mitigate it. The chemistry of the salt changes over time and the performance impacts of reactor behavior must be understood.
5 - Fission Products and Salt
Bret Kugelmass: Do fission products interact with the salt in molten salt reactors to form new compounds?
Lou Qualls: Fission products are put into the salt as the as the reactor runs and they are tracked over time and grouped into classes. Noble gases come out of the system because they don’t react with anything and a system in the reactor lets them escape. Some fission products react with the salt, the fluoride or chloride, and can become soluble in the system. Soluble products have an impact on reactor behavior, but as more soluble fission products are in the salt, there is less room in the salt for uranium. A solid can be formed within the liquid salt and can nucleate and begin to grow. This same particle could end up in a filter somewhere, leaving the salt but still in the system. Other things can stick to the wall, such as noble metals that don’t react or create salt solutions. This may disrupt the flow, impact the dose in the area, or put a heat load on the component that is serious enough to worry about getting over temperature if not properly cooled.
6 - Design of Molten Salt Reactors
Bret Kugelmass: What are the impacts of a hot spot in a molten salt reactor?
Lou Qualls: Hot spots could create a problem with a specific component. If maintenance has to be done on that component, there is no longer an easy way to access it to do deposits stuck on the wall. Monitoring and maintenance of a molten salt reactor is going to have challenges because of the distributed dose that is around the plant, not just in the fuel in the core underwater. Molten salt reactors don’t have a water pool above them so there must be different ways to shield and access. Camera systems that are tolerant to high doses of radiation and temperature must fit into the primary system. Components can be designed to last the lifetime of the plant or they can be designed to be replaced if they were not going to last the lifetime of the plant. This must be a thoughtful upfront design decision. The normal nuclear business thinks of everything as fixed and permanent, but molten salt reactors are more like a steam plant in which it is rebuilt over the span of its lifetime. The logistics of getting into the molten salt reactor business are challenges. Getting salts fabricated, transported, irradiated must be thought about. Supply chain on the front end must be created to make pumps and heat exchanges for molten salt reactors and have different design rules because of higher temperatures. This information must be shared with the NRC (Nuclear Regulatory Commission) and developers to get it into the design real-time. The nuclear business is in a difficult spot right now and needs a change. Evolutionary change is not always healthy; a revolutionary change is needed and molten salt can bring that to nuclear.
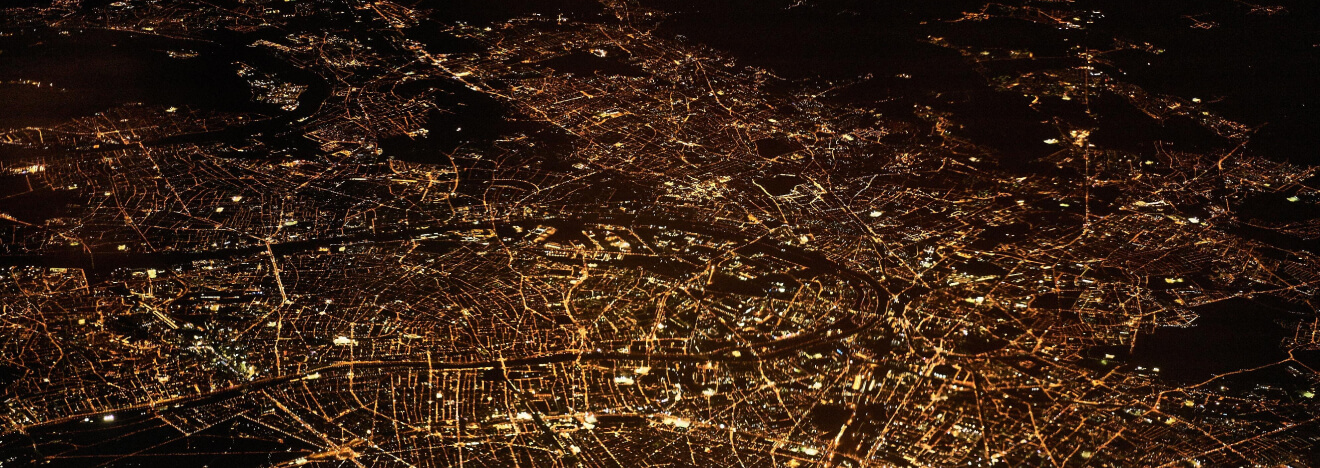
Sign up for our newsletter
No results found
Please try different keywords