TITANS OF NUCLEAR
A podcast featuring interviews with experts across technology, industry, economics, policy and more.
Latest Episode

1) The beginning of Brian’s career and his time in the United States Navy as a diver, as well as what drew him to engineering and nuclear
2) Brian’s initial journey to Oregon State and all of the research projects he’s had a hand in since then
3) Fostering a passion for nuclear in the next generation of nuclear engineers and why the researchers as just as important as the research itself
4) What challenges and successes the nuclear industry will face in the coming years and how to form your individual opinion on nuclear energy

1) Mark Lewis’ career trajectory in finance and energy as Head of Global Business Development at General Electric and Chief Business Officer at Tri Alpha Energy
2) How Tri Alpha Energy radiation-free fuel path uses hydrogen and boron for nuclear fusion
3) Driven by natural disasters, the focus on micro grids and the need for grid resiliency and lower carbon energy
4) Tri Alpha Energy’s use of plasma physics and accelerator physics to stabilize plasma in nuclear fusion
At this time we are still producing show notes for this episode. Please check back again at a future date.
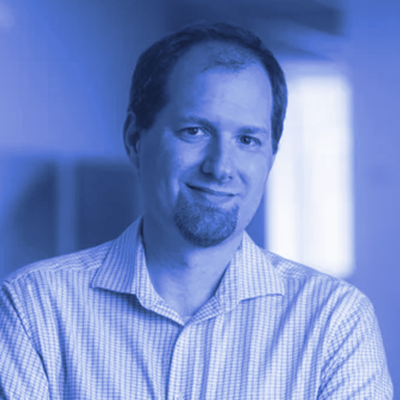
1) Bob’s background in fusion and personal journey in the nuclear industry
2) Superconductors, their applications, and how Bob has significantly contributed to their productization
3) Why only 3 of over 100,000 superconductors have been commercialized
4) Bob’s mission to expedite superconductivity commercialization so that it can be deployed globally
At this time we are still producing show notes for this episode. Please check back again at a future date.

1) Dennis’ background and personal journey in the nuclear industry
2) Why heat and density are crucial to successful fusion and the material challenges involved
3) Design challenges surrounding fusion systems including pumps and their inability to distinguish between helium and unburned fuel
4) Dennis’ mission to change the global nuclear industry through his work at MIT to make fusion economically viable
1 -
Bret Kugelmass: Tell me about your backstory.
Dennis Whyte: Dennis Whyte grew up in a remote location in Saskatchewan, Canada. He loved math and science and always knew that he wanted to be a scientist involved in exploration. Whyte was interested in fusion early on, reading Popular Science papers and writing his physics term paper on the topic. He studied engineering physics at the University of Saskatchewan, which had a small plasma program, focusing on mostly atmospheric plasmas. During his last semester of his senior year, Whyte got a recommendation from a professor to do graduate work at a new fusion lab outside of Montreal. A tokamak is a donut-shaped chamber with magnets, which is one of the most popular devices used to study fusion and make fusion happen. This program was hosted at an industrial laboratory, Hydro-Québec’s research facility. The plasma physics discipline is most commonly associated with fusion. Plasma is a phase of matter. If gases are heated past 5,000-10,000 degrees, they become a plasma. The center of the Sun if 15 million degrees and is where fusion occurs. Fission is when a neutron splits apart an unstable atom, like uranium, and releases energy. Fusion happens when the lightest nuclei, hydrogen and its isotopes are forced to create helium by getting the nuclei close to each other. Both have positive charges, so they repel each other and must be accelerated and slammed into a target. The probability of fusion occuring is very small.
2 -
Bret Kugelmass: If two particles are not hot enough or fast enough, do they just dissipate energy instead of fusing together?
Dennis Whyte: Yes, every particle has very high average kinetic energy. The center of a star is 15-20 million degrees, which is hot enough that the particles exchange kinetic energy. On Earth, a temperature of 100 million degrees is required to make fusion. A different fusion process is used than that of stars. Stars are extraordinarily good containers of energy and acts as an enormous gravitational container. When a fusion reaction occurs in the center of the Sun, huge amounts of energy are released, which eventually leaves as light. Stars don’t make a lot of power for how big they are, averating one watt for one cubic meter in the Sun. On Earth, the goal is many millions of watts per cubic meter. The power density is the most attractive part of fusion. The relative environmental footprint is reduced and in the end becomes a challenge of low power density sources, like renewables. Within a week of being in a research lab, Dennis Whyte knew it was what he was going to do for the rest of his life. Whyte received a national grant from the Canadian government as a post-doc which encouraged him to go outside Canada to gain experience. He went to San Diego where the General Atomics experiment D3D on a tokamak was ongoing. Canada then cancelled their fusion program, so Whyte stayed in the United States. He wanted to have the intellectual freedom that comes with being an academic, to pursue his own ideas in a way that wasn’t possible as a more structured research scientist. He wasn’t as motivated about the student at first, but discovered he loved teaching. Becoming a teacher made Whyte a better researcher. The exercise of explaining the fundamentals to students led to ideas and turned into big projects. Whyte spent four years at the University of Wisconsin, but he missed being in a bigger team and working on the applied part of research. Whyte moved to MIT because of its Plasma Science & Fusion Center, of which he is now the director. Whyte has been at MIT for 12 years.
3 -
Bret Kugelmass: What did the plasma program look like when you first got to MIT versus what it looks like today?
Dennis Whyte: The specialty of MIT’s fusion program has always been built around magnet technology. Because of the expertise present, they had the ability to make magnetic fields that were much stronger than other laboratories were considering. By some basic arguments, they made the device smaller, which became Alcator. When Dennis Whyte first went to MIT, Alcator C-Mod was the third iteration of the fusion experiment. The focus of the program was education and training. It was solely funded through the Department of Energy and the program stopped a couple years ago. On the last day of its operation, it set a world record for fusion performance by surpassing 2 atmospheres of pressure in a plasma that was significantly hotter than the center of the sun. Doubling the pressure seen in other devices quadruples the energy density, making it more economical. Magnetic fields exert a magnetostatic pressure that is counteracting or containing the plasma. The material of challenge for the hot part of the fusion is that there can’t be any materials around. The plasma is tricked into thinking it’s not on Earth by creating a vacuum inside the donut and turning on the magnetic field. Nothing physically contacts the plasma, otherwise the plasma would become cold.
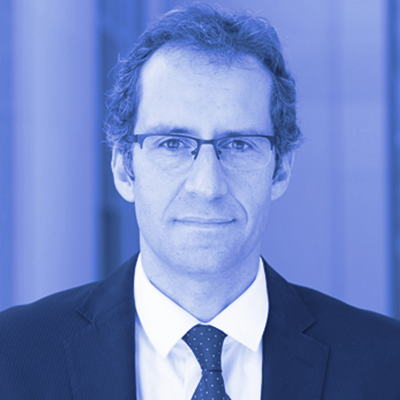
1) Jacopo’s background in techno-economic analysis and personal journey in the nuclear industry and work at the Idaho National Laboratory
2) Jacopo’s contributions to MIT Energy Initiative’s recent report, “The Future of Nuclear Energy in a Carbon-Constrained World”
3) The benefits of assembling several microreactors to take advantage of economies of multiple versus economics of scale of size
4) Shipyard building and how Jacopo’s research at MIT works to reduce construction costs
1 - International Pursuit of a Career in Nuclear
Bret Kugelmass: Tell me about yourself.
Jacopo Buongiorno: Jacopo Buongiorno grew up in Italy and came to MIT in the U.S. for his doctorate, which he completed in 2000. He worked for four years at the Idaho National Lab (INL), which was then called the Idaho Engineering and Environmental Laboratories. In 2004. Buongiorno returned to MIT to join the faculty of the nuclear science and engineering department. His undergraduate nuclear engineering degree was completed in Italy at Politecnico di Milano and Buongiorno felt the professional opportunities were the best in the U.S. In 1986, the accident at Chernobyl happened and Italy fought against nuclear in 1987. Buongiorno enrolled in his first nuclear program in 1990, attracted by the diversity of topics and subjects that he would be exposed to since nuclear is inherently multidisciplinary. It was clear to Buongiorno that nuclear held great promise in terms of satisfying the world’s energy needs and being overall safe and clean. While at INL, Buongiorno was heavily involved on a lead bismuth cooled fast reactor, a follow-up project of his thesis project. Lead bismuth has benign behavior in interactions with other materials and has a very good natural circulation potential. The lead bismuth technology was developed and deployed in the Soviet Union, used for a class of submarines. Without any oxygen, the lead bismuth attacks metals. A little bit of oxygen creates a protective layer, but too much oxygen creates a lead and bismuth oxide sludge.
2 - Nuclear Science and Engineering at MIT
Bret Kugelmass: What specifically where you focusing on during your thesis at MIT and later at Idaho National Labs?
Jacopo Buongiorno: Jacopo Buongiorno was working mostly on the natural circulation behavior of the lead bismuth fast reactor system during postulated abnormal events. During these events, the normal heat removal path through the steam generators or the intermediate heat exchangers is lost and decay heat must be removed another way. One idea looked at was the implementation of RVACS (reactor vessel auxiliary cooling system) in lead bismuth reactors, which was developed for sodium reactors. The thermal capacity of the coolant can be leveraged to delay the rise in temperature inside the reactor, but the decay heat lingers and the system will accumulate too much energy. There are ways to remove decay heat in a reliable and robust way without relying on active systems, such as utilizing natural circulation. The desire to teach as always in the back of Buongiorno’s mind and he enjoyed the teaching assistant experience he had while at MIT. Buongiorno was attracted to the freedom of academia and the renewal that comes with new classes of students. He has been teaching for 14 years and is now the associate head of the nuclear department. The first “Future of Nuclear Power” report was conducted in 2003, led by professors John Deutch and Ernie Moniz. The landscape for nuclear, and energy in general, 15 years ago was much different than today. People were excited about a potential upcoming nuclear renaissance, fossil fuels were relatively expensive, and wind and solar were on the radar, but not as credible as they are now. Two years ago, the nuclear department at MIT decided to launch a new study on the future of nuclear.
3 - The Future of Nuclear Energy in a Carbon-Constrained World
Bret Kugelmass: What are initial conversations like to rally support for a study on the future of nuclear?
Jacopo Buongiorno: Conversations start at the individual level, one-on-one, with colleagues to talk about how things have changed for nuclear. The new report is called “The Future of Nuclear Energy in a Carbon-Constrained World”. Electricity and power are the primary products of nuclear energy, but are not the only products. The report focuses heavily on the power sector, but also on non-electricity applications. MIT wanted to look at what role nuclear could play as the world seeks to decarbonize its energy systems. A region-specific analysis must be done because the input in the study is highly dependent on the region. Nuclear plant construction is built at a higher cost in the U.S. and at a lower cost in Korea, China, India, and Japan. Some ideas and innovations have been identified that could lower the cost of nuclear. The largest cost of nuclear is in the construction of the plant itself. If nuclear is in the business of reducing costs, the way the plant is built and delivered must be reconsidered. Just changing the coolant or the fuel for the sake of innovation will not reduce cost. A small reduction in construction costs could make a big difference in the capacity of nuclear that could be deployed. In order to get solid cost estimates for nuclear, or other complex technology, one must proceed into higher level design cost estimates.
4 - Construction Costs of Nuclear Plants
Bret Kugelmass: Why are construction managers doubling their costs in their revisions to original estimates for nuclear power plant construction?
Jacopo Buongiorno: Predicting the cost and execution of the project has been a spectacular failure in the nuclear power plant industry. The AP-1000 was designed and received a design certification, but the type and amount of design that is done for licensing is fundamentally different than the detailed design needed for construction. There was a commercial pressure within the companies to start and external constraints such as loan guarantees or production credits, causing companies to rush into the construction process. The industry in the U.S. and in Europe is proficient at operating the plants, but constructing the plants is a skill that must be rebuilt. The total cost of delivering a nuclear power plant includes buying the components from a supplier, financing, site preparation, civil works and labor, engineering costs, including design and licensing, and so-called owner’s cost, which includes the cost of the land or other things. The direct cost of equipment is 20-25% of the cost of nuclear plants. The cost of the installation, including heat sink, excavation, and preparation is about 50% of the cost. The implications of this finding are that different areas need innovation, such as concrete structures, excavation, or design. MIT’s report identifies three or four innovations outside the traditional realm of nuclear engineering which have the potential to reduce the cost because they tackle the question of how the plant is built, not what specifically is built.
5 - Government’s Role in Nuclear Energy Development
Bret Kugelmass: Who is the audience of MIT’s report “The Future of Nuclear Energy in a Carbon-Constrained World”?
Jacopo Buongiorno: “The Future of Nuclear Energy in a Carbon-Constrained World” has a diverse set of audiences. The first chapter, aimed at energy policy planners, looks at the role that nuclear power can play in a decarbonized world so people can recognize that nuclear has to be part of the picture to solve global warming and reduce emissions. If nuclear is excluded, solar and wind must be built out enormously, with storage. The government has to play a role in supporting and providing R&D that targets such innovation. It also needs to put all low-carbon energy technologies on the same plane, instead of a potpourri of subsidies for certain technologies. Ideally, a carbon tax would be the best solution since it is technology-neutral, but it doesn’t have the political support. The government also plays a role in facilitating and promoting the development of new nuclear technology, potentially by providing sites where advanced reactors or small modular reactors (SMR) could be deployed. This class of advanced reactor technologies does not require anything new in the regulatory space, but some less mature Generation IV systems may require 25-30 years in the traditionally regulatory space. MIT looked at a way to potentially accelerate this process by combining the engineering demonstration and the full-sale demonstration into one machine, meaning the first machine must be built at full-scale. The prototype rule in the Nuclear Regulatory Commission (NRC) could allow the design and construction of the demonstration machine with a very large built in margin. Once the prototype is built, a series of incremental tests would give and create confidence in the system at each step. Once the program is done, the hope is that the NRC will approve the demonstration of technology and allow the plant to be first-of-a-kind.
6 - Modular Reactor Construction
Bret Kugelmass: Did MIT talk to the Nuclear Regulatory Commission about innovation in the regulatory system?
Jacopo Buongiorno: MIT talked to the Nuclear Regulatory Commission (NRC) during the development of the report. Many people thought there were multiple showstoppers on the thought of innovation in the regulatory system. Innovation in reactors may not be in new materials, but perhaps in a new layout or different concept in deployment of the machines. The idea of modular construction, or shipyard construction, has merit. The business of modularity has been on everybody’s mind in the nuclear community. MIT looked at how the modular construction approach has been a game-changer in other industries, since there is evidence that is has reduced cost. Chemical plants are by-and-large build modulary and assembled on-site. Virginia-class nuclear submarines have been built more and more modularly, which has enabled a lower cost and more compressed schedule. After a study on the ABWR in Japan, modular construction made a difference on the order of 20%. In a separate effort from the study, MIT looked at building modular reactors in shipyards and integrating them into platforms. Buongiorno continues to teach classes at MIT, but has changed direction in his research space. He still continues to work on a couple of projects in his traditional area of thermohydraulics and boiling heat transfer, but Buongiorno has started new projects that address the question of how to integrate innovations in construction into new reactors. One new project is with Tokyo Electric Power Company (TEPCO) who is looking at the Japan nuclear system of the future. After Fukushima, there’s a lot of uncertainty about the future of nuclear in Japan, so this project is an opportunity to change the debate on nuclear into something more positive.

1) Mark’s background in biology and personal journey in the nuclear industry
2) The decommissioning of Rocky Flats Plant, a nuclear weapons production facility that now serves as a model for other decommissioning projects
3) Mark’s work with British Nuclear Fuels Limited and then later as Chief Nuclear Officer for Magnox South
4) The benefits of SMRs and Canada Nuclear Lab’s mission to facilitate global implementation
1 - Early Days in Commercial Nuclear
Bret Kugelmass: Where did you first familiarize yourself with nuclear?
Mark Lesinski: Mark Lesinski grew up on Grand Island in the U.S. He has a biology background and studied at the University of Buffalo, where he became interested in radiation science. Lesinski started working at a facility making radioactive sources for industry. He later went to graduate school for health physics and moved on to commercial nuclear from there. One of the problems with radiation is that humans cannot sense it, so instruments had to be invented to sample the environment. When Lesinski transitioned to nuclear power, he got into the operations side of the industry and, later, the project side. By having a different viewpoint, one can try to do a better job of empathizing with the challenges each group is facing. Lesinski cut his teeth on boiling water reactor (BWR) doing modifications and pipe replacements. Issues with intergranular stress corrosion cracking was affecting in the recirculation piping and a whole house change-out was needed to keep asset going. The change-out was never intended in the design, so methods of getting in to replace the pipes had to be determined and executed. When Lesinski moved into the pressurized water reactor (PWR) side of the business, they began to replace steam generators. One of the solutions was to cut a hole in the side of the containment building, remove the faulty component, put a new one in, and repair the hole per standard so it can still be pressurized and act as containment.
2 - Nuclear Decommissioning in the US and the UK
Bret Kugelmass: What steps did you take to round out your nuclear experience?
Mark Lesinski: Mark Lesinski migrated into the decommissioning side because, as many repair activities that took place, some plants just reached the end of their life. His first decommissioning was at Big Rock Point, a small boiling water reactor (BWR) in Charlevoix, Michigan. Lesinski then went to Rocky Flats, a very successful weapons facility in the Department of Energy. During decommissioning, about 1,000 buildings had to be taken down and a lot was learned about environmental cleanup. Rocky Flats is pointed at as a model to the industry for cleanup. As he left Rocky Flats, Lesinski joined a consortium to look at decommissioning a sodium reactor in the UK, which they did not win. The Nuclear Decommissioning Authority (NDA) was going to take the assets of the first reactors in the UK and bundle that with 18 other different sites under one organization to be managed and to establish a government-owned, contractor-operated model. Lesinski went over to work with British Nuclear Fuels as they were divesting and the NDA came in to set up contracts. He started out as a transition manager, quickly becoming the site director at Hinkley, the chief nuclear officer for Magnox South, and later the managing director. Lesinski joined the NDA as the chief operating officer for all 18 sites across the UK. He had an understanding of how the team had to perform and how the work would be completed compliantly and safely. Lesinski showed that great safety statistics could be achieved at the same time as reaching milestones.
3 - Canadian Nuclear Laboratories
Bret Kugelmass: How did you make sure that best practices were shared?
Mark Lesinski: Successes and failures were shared and this set of decommissioning missions is still is ongoing. Mark Lesinski spent 10 years in the UK, the last of which he spent working for Rolls Royce. An opportunity came up at Canadian Nuclear Laboratories (CNL), which includes multiple different sites, many of which will go away after cleanup. Whiteshell in Manitoba is a research site that has prototypes and hot cells, but it currently undergoing decommissioning. The Port Hope Area Initiative in Ontario deals with tailings from the facilities doing separations in the harbor a long time ago. The tailings from the process of separating radium and uranium were spread around the community. After many years of negotiation, the Canadian government made an agreement with the municipalities to clean up the site. Two big surface waste facilities are being created to store the material safely into the future. CNL has a number of prototype reactors, including NPD (Nuclear Power Demonstration), which was the first CANDU to get online. Douglas Point, on the Bruce property, is another closed CNL liability and Gentilly is another closed demonstration reactor in Quebec. Heavy water is an asset, so CNL sells it to a lot of commercial operations and it must be stored as reactors are drained. New Brunswick is a facility working on process cybersecurity, including grid control and reactor control. Chalk River is where CANDU was born and is where the second sustained criticality occurred after Enrico Fermi’s work in Detroit. Chalk River was also Canada’s contribution to the Manhattan Project, but Canada took a very deliberate step away from the weapons side, putting the country in a position in which they can be a good arbiter for international nuclear work.
4 - Impact of Chalk River Laboratories
Bret Kugelmass: Tell me about Chalk River’s role in all things nuclear.
Mark Lesinski: There is so much more to nuclear that is not weapons, however, when people talk about nuclear, they see a mushroom cloud. Isotopes, low-carbon energy, industry applications, and understanding materials are all aspects of nuclear that people miss. Canada is a great example of the positive aspects of nuclear. The costs for making isotopes were being borne by the Canadian taxpayer and a decision was made that Canada would not subsidize the product. About a half billion patients had scans from the moly-99 that was created in reactors at Chalk River. About a half billion patients whose tumors were treated via the cobalt-60 that was also created at Chalk River. When Mark Lesinski got into nuclear, the industry was already starting to retreat and didn’t do the outreach needed so that people would understand the technology. Lesinski and his team arrived at Chalk River in 2015. The Canadian government decided to restructure and do some divestiture of Atomic Energy Canada Limited (AECL). At the time, AECL had the CANDU design and was trying to sell the reactors and make isotopes as a government organization. They sold off the CANDU portion to SNC-Lavalin, who are now the ones pushing and promoting the new design. The laboratory had a lot of liabilities because there has been a lot of waste issues and, in the early days of nuclear, they didn’t know what to do with waste. The industry knows how to deal with the waste now, but it has become a political and social issue and is no longer a technical issue. A government-owned, contractor-operated approach was adopted for the laboratories with the missions being clean up of waste and also an investment of $1.2 billion into new buildings and facilities to have new missions and be relevant in the world.
5 - Small Modular Reactors in Canada
Bret Kugelmass: What is nuclear’s role in baseload power?
Mark Lesinski: Renewables are really good up to point, until the cost is doubled because the amount of solar panels and wind turbines would need to be doubled to provide baseload power. One of the missions at Canadian Nuclear Laboratories (CNL) is small modular reactors (SMR). The northern part of Canada is very sparsely populated, but there are many communities that exist whose quality of life is suffering. They currently get electricity from diesel that is transported up via the ice roads or flying it in, making it incredibly expensive and accelerating their environmental problems. Canada has a huge domestic need for another solution. They are also looking at wind and solar, but during a cold, long winter night, there is no sun and often no wind, making it so they will never be able to survive on renewables. Industry has to work hard to get people to understand the technology and listen to any issues they may have. Canada has a nuclear regulatory system that is looked at as one of the models to be replicated in other countries because they protect the public, but are also technology-agnostic. The agility of the regulator can help make SMR deployment happen. In order to make a nuclear reactor, it requires a huge supply chain. Canada has the biggest refurbishment projects going on in the whole world of nuclear right now, which requires a supply chain. The schedule for completion of Canadian refurbishment and deployment goals for SMR’s match up beautifully.
6 - Canada’s Keys to Success in Nuclear
Bret Kugelmass: Can the nuclear decommissioning teams transition to become the manufacturing workforce for the next generation of reactors?
Mark Lesinski: The teams working on nuclear decommissioning can transition to become the manufacturing workforce for the next generation of reactors. Canada is the only place this is happening around the world. There must be a workforce that understands how to complete the work and be successful at it. There is a whole litany of reactor designs that were looked at at the Canadian Nuclear Laboratories (CNL), including CANDU and the SLOWPOKE reactor, one of the first small modular reactors (SMR). Between Chalk River and Whiteshell, CNL has 22 locations where demonstration reactors could be put in. The communities understand nuclear and understand how important it is to issues being faced in the world. A good regulator can back CNL up and the labs have all the supporting facilities to support a demonstration. CNL’s researchers understand how to work through issues, where it is related to fuels, materials, biological effects, or any other line of expertise. Mark Lesinski was attracted to nuclear out of curiosity, but discovered a well-run industry that is doing amazing work. Through the millenia, humans have gone to more and more concentrated energy, so nuclear made sense from a power side and had all other benefits. Nuclear is part of the baseload solution. The industry must get through to the public about what is going on in nuclear and how nuclear knows how to deal with issues. Nuclear is a big piece of the puzzle for quality of life in the future.
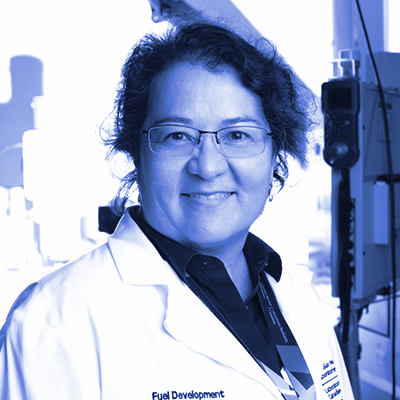
1) Rosaura’s interest in nuclear from a young age and her journey through the industry
2) A deep dive into the importance of ceramics processing in regards to creating nuclear fuel
3) Challenges associated with fuel processing and manufacturing and how Canada Nuclear Labs is working to overcome them
4) The importance of waste management technology to nuclear success
1 - Impact of Temperature on Materials
Bret Kugelmass: Tell me about your backstory.
Rosaura Ham-Su: Rosaura Ham-Su was born and raised in Mexico City, leaving for Canada in her early 20’s. Her family lived in small towns without electricity and Ham-Su was able to see the amount of effort required to get water into the house without electricity and a pump. The type of pollution that was generated in order to get energy stuck with Ham-Su and she was always fascinated by how energy is produced and what is left behind. She studied engineering physics in Mexico at La Universidad Iberoamericana and received her PhD in material science from McMaster University. The focus of Ham-Su’s work was the processing of ceramic material. The way any material is processed as an impact on its behavior and properties afterward. Ceramics were looked at for structural applications to be used at high temperatures. Creep properties change a lot depending on how it was processed. When uranium is extracted from the ground, it has uranium, oxygen, and other things, so it must go through a separation process to go to different purifications to produce the wanted uranium. Metallic uranium is often produced and brought back to uranium oxide. When uranium oxide is put at a very high temperature in air, it will oxide more. To make a pellet UO2, the powder must be compressed and put in the sintering process in a hydrogen atmosphere.
2 - Magnetic Shape Memory Alloys
Bret Kugelmass: How are nuclear ceramics made?
Rosaura Ham-Su: Ceramics start with a very fine powder that is pressed into granules. Granules need to be big enough so gravity will take over their behavior. The granules get pressed again and becomes a green pellet, which is not ready yet. The green pellet goes through the sintering process in a hydrogen atmosphere and comes out 95% dense with 5% pores. This solid ceramic finished product is used for most nuclear reactors. Rosaura Ham-Su has always been focused on how the processing affects materials and how processing can be used to get different products. One of her jobs was to figure out how to make ceramic armour. Ham-Su went to work for Canada’s Department of National Defence to work on magnetic shape memory alloys, which change shape based on magnetic field. Their shape can also be changed to create a different magnetic field. This single-phase alloy was nickel manganese gallium. Their magnetic domains align with a crystal structure and are able to twin, in which a crystal changes shape very quickly. A changing magnetic field can create a current in the right conditions. These energy harvesting devices were to be used to power buoys that detect activity along the expansive Canadian coast.
3 - Recycling Spent Nuclear Fuel
Bret Kugelmass: Tell me about some of the challenges in fuel processing and development.
Rosaura Ham-Su: Fuel development is dedicated to making fuel more proliferation efficient, more efficient, more recyclable, and in general, more sustainable. The economics landscape changes depending on what’s happening around the world. Some concepts are worked in the lab that may not have the economics in the moment, but are still worked on in anticipation of changes that it will bring to the nuclear industry and could change the landscape of economics. Recycling of fuel has been worked on by the physicists in the lab for a long time and always starts with physics saying something is possible. Spent nuclear fuel has many interesting elements in it that could be used for radioisotopes for cancer treatments or more energy, but it is very radioactive. The conditions of the new fuel to produce more electricity are given by the physicists and the next challenge is to make it into a shape to demonstrate that it can be done. Fuel is not an infinite resource; even though there is enough for the reactors now, it is a source coming out of the ground and eventually it will be depleted. The concerns about nuclear waste are always the plutonium or the daughters of plutonium. When plutonium is removed from the fuel, the problems of the waste change in timescale by orders of magnitude. Part of the driver of using plutonium is to use it and not create any more. Some isotopes in spent fuel are useful, but some might be better destroyed and the creation of nuclear weapons is also a concern.
4 - New Nuclear Fuel Development
Bret Kugelmass: How detailed is the characterization of spent fuel?
Rosaura Ham-Su: Historically, every radioactive isotope is characterized in spent fuel. There are other things created that could be nonradioactive, but are not as well characterized. In long term storage, the fuel could create something that is corrosive, but not radioactive. One of the drivers for new fuels is to be safer, such as accident tolerant fuels (ATF). New technologies like additive manufacturing may also contribute to fuel development. The middle of a fuel pellet gets to approximately 2,000 degrees Celsius. Ages ago, the Russians designed a fuel that was hollow. The Canadian Nuclear Laboratories designed a fuel that was internally cooled where the water went through the pellet to remove the heat. One of the big criticisms for that fuel design was that it would be difficult to manufacture, but 3D printing may change that story. There are lots of interesting concepts in fuel development. Some concepts are used for isotope targets instead of producing energy. The elements important for the target need to be separated so they can be used for medical applications.
5 - Fuel Development at CNL
Bret Kugelmass: How does the ratio between Pu-239 and Pu-240 change over the life of the fuel within the reactor?
Rosaura Ham-Su: Every reactor is different, but the ratio is dependent on the residence time of that fuel in a given reactor. A steady state can be achieved based on the burnup and the physics of the reactor. In water reactors, there is a residence time that is ideal for getting energy out and changing the ratio. Civilian plutonium has more plutonium-240 and military-grade plutonium has more plutonium-239. Rosaura Ham-Su currently has a project in 3D printing at Canadian Nuclear Laboratories (CNL) and just finished up a big project on thorium. Thorium can be used to deplete plutonium from the world and is a plutonium consumer. Thorium does produce u-233, which has a strong gamma signal. Part of the concern with plutonium is that, when it is pure and young, it doesn’t have a gamma signal so it is hard to detect. There are different proliferation concerns with u-233. Thorium is a lot more abundant that uranium and can also be used to recycle fuel. It can be used in pellets or in molten salt. CNL goes very quickly into producing electricity, but also has research reactors that use different types of fuel, such as liquid metal. The advantage of uranium-moly fuel instead of uranium silicide is that it has a higher density. This allows low enriched uranium, which has less uranium-235, to power a research reactor instead of high enriched uranium. The marriage between fuel design and spent fuel management is very important. Current reactors were designed thinking about the production of electricity and the waste or spent fuel management was something to be thought about afterwards. As new reactors with new fuels are built, the whole fuel cycle will have to be thought about and well-planned to avoid leaving another generation with this problem.
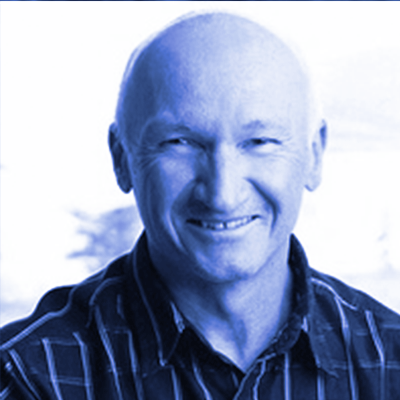
1) Metin’s background in mechanical engineering and personal journey in the nuclear industry
2) A deep dive into CANDU (Canada Deuterium Uranium) reactors
3) How technologies and designs within supercritical coal plants could potentially be recycled to create supercritical nuclear plants
4) The Pan-Canadian SMR Initiative and its mission to facilitate global implementation
1 - Vibrations of Steam Generator Tubes
Bret Kugelmass: Where did your career in nuclear begin?
Metin Yetisir: Metin Yetisir is a mechanical engineer from Turkey who came to Canada 35 years ago to pursue his Master’s at McMaster University focused on vibrations of steam generator tubes. The nuclear application of his studies was looking into vibrations of steam generator tubes in nuclear reactors. Steam generators, also called boilers, transfer heat that’s generated in the reactor core to the secondary side fluid which turns into steam. The steam is used in turbines to produce electricity. During the process, the secondary side saturation pressure is lower than the primary side, causing different boiling temperatures. The secondary flow that is flowing through the steam generators are going around the tube, which the primary side flow is inside the tubes. The flow velocities for the secondary flow is high and it expands causing turbulence, exciting the tubes to vibrate. Fluid elastic instability happens when the vibration pattern introduced by flow happens at a frequency that matches the natural frequency of the tubes. Turbulent excitation causes vibration at low levels, which causes rubbing and impacting issues at the support locations. When the system becomes fluid elastically unstable, the tubes might rub at the mid-span and collide with each other. All the energy in the tubes causes failure fairly quickly.
2 - Heat Transfer in Heavy Water Reactors
Bret Kugelmass: Tell me about your studies in thermal performance and degradation.
Metin Yetisir: Steam generators have a mechanical aspect, which is vibrations, and a fluid aspect. Metin Yetisir worked in flow-induced vibrations, which required an understanding of fluid flow and mechanical aspects. Yetisir started looking into heat transfer because the steam generator performance affects the performance of the entire power plant. If heat cannot be extracted from the primary side efficiently, the power is not effectively being transferred to the secondary side. In CANDU reactors, this brings the primary side temperature and energy level up in the reactor. When the feed water flow is coming from the turbine side, impurities in the water settles on heat exchanger tubes and creates a layer of crud. This crud could be particulates or dissolved material. There are a lot of advantages to using heavy water since it is a great moderator. In the supercritical water reactor concept, a heavy water moderator is a last resort cooling system. If all power is lost and coolant in the supercritical reactor, the heavy water that is where the fuel channels are sitting provides additional cooling. In pressurized water reactors (PWR), light water is functioning as a coolant and moderator at the same time. In CANDU reactors, a heavy water coolant is located on the primary side, in the fuel channels. The fuel channels are sitting in a vessel which is also filled with heavy water, where it acts as a moderator. Having two separate systems means that, if the primary side coolant is lost, there is still a moderator sitting there as an additional source of coolant that could be used.
3 - Supercritical Water Reactor Concept
Bret Kugelmass: What are the benefits of using water as a coolant and a moderator?
Metin Yetisir: Water-cooled reactor safety systems come down to how much water is available, how quickly it can go into the reactor core, and how quickly it can remove heat. CANDU reactors could be easily modified so everything is based on passive concepts. Passive systems are safer because they don’t rely on components that can break and component performance does not need to be justified. There are advantages of using active systems using pumps. These systems can inject water faster and the speed of it can be controlled, but they do not have the reliability. A passive system has very few components. Active systems have a lot of moving parts on components and a lot of time must be spent to ensure everything is fit for service. Passive systems tend to require a larger space. The trend is moving from active systems to passive systems. Small modular reactors (SMR) aim to be safer and operate with a lot less staff, to make them more economical. Supercritical phase is a single phases that happens past critical point, a particular pressure and temperature point. In supercritical condition, there are clusters of molecules that move like gases but behave like liquids. The density change between the liquid phases and the gas phases is not as big as it is at lower pressures. In the supercritical water reactor concept, there is a fuel channel with a central flow tube that brings the supercritical water in a dense form. As it comes to the bottom, it turns up and goes up through the fuel elements and starts becoming gas-like. By the time it reaches the top of the fuel channel, it has a ten times lower density than its initial density coming down the flow tube. Efficiency is directly related to the steam temperature. There are about 600 supercritical fossil plants available in the world, which is sometimes referred to as “clean coal” due to lower emissions and higher efficiencies.
4 - Advanced Reactor Technology at CNL
Bret Kugelmass: Why don’t any giant supercritical coal companies invest in making a supercritical nuclear power plant?
Metin Yetisir: Normally, the design for a supercritical coal plant is done but the rest of the equipment is purchased from manufacturers who do the turbine and the secondary side equipment. Those technologies are typically not coupled. Metin Yetisir runs the advanced reactor technology group at Canadian Nuclear Laboratories (CNL). Yetisir got involved with supercritical research reactor work in 2009 and they came up with a concept called Canadian Supercritical Water Reactor. The small modular reactors (SMR) are being talked about a lot in Canada and use Generation IV advanced reactor technologies, such as sodium-cooled, molten salt, gas-cooled, and liquid reactor concepts. Labs are shifting gears towards addressing those technology needs. CNL used to be solely focused on CANDU reactors, but is now looking at all reactor technologies that could help the industry within their capabilities. It takes a long time to become really good at all the different technologies. Tritium production and management is an issue in CANDU reactors because of the heavy water. When heavy water is exposed to neutrons, it produces tritium. It is easier to produce tritium from heavy water reactors than light water reactors. One of the isotopes of lithium, which is part of the molten salt reactor, converts to tritium when exposed to neutron bombardment.
5 - Pan-Canadian Small Modular Reactor Roadmap
Bret Kugelmass: How are looking at different advanced nuclear systems and how we understand their inherent challenges?
Metin Yetisir: The requirements for building new nuclear reactors are based on the needs they are being built for and the timelines considered. They may be built to get rid of or minimize nuclear waste. However, all the technologies could be considered. It is unknown, until after getting a lot of experience, which technology will work better. By selecting one favorite technology and dropping the others, there may be missed opportunities to find the best one. There is an ongoing effort called the Pan-Canadian Small Modular Reactor (SMR) Initiative. This initiative brings everyone interested in SMR’s together, including four provincial governments and multiple utilities. The goal of the initiative is to come up with a roadmap towards hows to make SMR’s possible in Canada and create the right environment for it to happen. Having a pan-Canadian approach makes SMR deployment more likely. One thing they are looking at is how the supply chain effect may impact the selection process. The SMR supply chain will be much easier to satisfy than a large reactor since many of the parts are smaller. Some of the issues may come with the fuel that is available, but the industry could mobilize fairly quickly to supply mechanical components. There may be issues with moving reactors to higher temperatures because sensors and monitoring systems may need to be reconsidered, but it provides an opportunity to come up with better techniques. When Metin Yetisir first went to Chalk River, he thought that all the interesting things in nuclear happened in the 1950’s. Many interesting concepts are coming back again and with new ideas. If SMR deployment happens, it will be great for Canada, the United States, and the rest of the world.
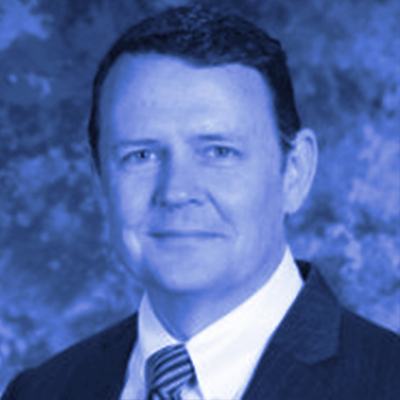
1) Corey’s background in nuclear engineering and personal journey from Los Alamos to Canada Nuclear Labs
2) Corey’s contributions to nuclear startup NuScale Power
3) Challenges in nuclear across India and Asia including infrastructure, training, and corruption
4) Canada’s new build market and the significant role it plays in the global industry
1 - Nuclear in Technology and Politics
Bret Kugelmass: What led to your interest in nuclear engineering?
Corey McDaniel: Corey McDaniel went to Purdue University on an ROTC Air Force scholarship which let him study anything he wanted to. He had an interest in engineering and physics which was inspired by a high school physics teacher. Nuclear was attractive to McDaniel as a mix of mechanical, civil, materials, electrical engineering and nuclear physics. A professor connected him with Los Alamos National Laboratory in his home state of New Mexico where he spent a summer internship doing thermohydraulic analysis. McDaniel wanted to work on space reactors, but the program was cancelled right before he graduated. This led him to probabilistic risk assessment (PRA). Some small modular reactors (SMR’s) are based largely on the technology that was developed for space nuclear. McDaniel has gone between working in purely technical environments, like at Los Alamos, and working on presidential campaigns. In 2005, he worked on the Energy Policy Act with Idaho Senator Larry Craig through the recommendation of his home state Senator, Pete Domenici. McDaniel wrote sections of the Act for next generation nuclear plants, clean energy, and addressing climate change through technology and loan guarantees. Different incentives could be used to get nuclear going again, such as standby support, production tax credits, and loan guarantees to send an overall environment to send a signal to the market that the government valued nuclear power.
2 - NuScale’s International Development
Bret Kugelmass: What changed from the times that nuclear technology could have been built easily?
Corey McDaniel: At one point in nuclear, the incentives were there and the environment was right for building nuclear technology. There are different reasons why the nuclear renaissance didn’t happen. The Energy Policy Act only included one provision that referenced shale and shale wasn’t on anyone’s radar. Before long, the price of natural gas plummeted and the financial crisis happened. Fukushima happened in March 2011 and nuclear’s new build path in the U.S. was extinguished. Corey McDanial got his Master’s degree at New Mexico in nuclear engineering, which at the time, focused on environmental studies of nuclear clean up. Idaho National Lab is the national nuclear laboratory. Technology in fracking for natural gas to bring the price down doesn’t mean nuclear loses and is not a net negative for the environment. There is no price on carbon today. Greenhouse gases should be addressed through more innovation and new technology. In nuclear, it’s not enough to be really safe and to do things very clean; the plants must be very efficient and there must be more innovation. Core McDaniel was involved early on with NuScale Power, joining in 2010. When he joined the company, McDaniel was living in India and NuScale had about 80 employees,just getting to tens of millions of dollars of investment. McDaniel was the director for international business development for NuScale out of Mumbai, reaching 14 different countries. There was an emerging nuclear market in Southeastern Asia countries, who were interested in small modular reactors (SMR’s) due to the smaller investment needed, the safety, and the lack of competition with natural gas.
3 - Nuclear Energy in India
Bret Kugelmass: How does nuclear energy fit in India?
Corey McDaniel: India’s infrastructure is one of the biggest challenges in the country’s implementation of nuclear power, in addition to challenges with worker training and corruption. There are places in Eastern India that have the coal, but the land is inaccessible due to a lack of permission from the people. This region would buy coal from Indonesia at a guaranteed price, reliably and of the right quality. All the nuclear in India makes up a very small percentage of the country’s power and a significant portion of the population does not have any grid electricity. The distributive model may work very well in India and has been used by solar and wind. The idea of a container shipped nuclear reactor is still a ways away, but the Canadian Nuclear Laboratories are working at Chalk River with venture capitalists and small startup companies to work towards this goal. In India and China, solar has been something that can be deployed rapidly with immediate power. Canada is particularly interested in its remote locations in the Great White North which typically fly in diesel and sometimes have no sun at all. India is looking to deploy more of their existing technology and advanced heavy water reactors. Throughout most of the developing world, there is interest in small modular reactors (SMR’s), but it is dependent upon what the market can bear.
4 - Falsification of Nuclear Records
Bret Kugelmass: Where did you go after your work at NuScale?
Corey McDaniel: After leaving NuScale, Corey McDaniel worked for a nuclear electrical supplier of Class 1E cables that supply the safety systems. If an accident happens, these cables have to survive the conditions of the accident. McDaniel delivered cables to the UAE, China, and Korea. One of the biggest scandals in nuclear when a Korean cable supplier falsified their qualifications for their cables. These cables that didn’t meet flame test qualifications were discovered already installed in reactors. The subsidiary that manufactured the cables dissolved. The falsification of equipment in Korea and other places is fairly common for non-safety related equipment, but this was the first time it was discovered for safety related equipment. Korea’s current government is not supportive of nuclear going forward, partly related to Fukushima and partly due to the scandals. In the 1960’s, the U.S. had issues with CFSI (counterfeit fraudulent suspect items) and Japan went through their own period as well. The cost to the company for having the reactors shut down for cable replacement was estimated at more than four billion dollars.
5 - Chalk River Laboratories
Bret Kugelmass: What brought you to Chalk River?
Corey McDaniel: Corey McDaniel was at Idaho National Labs in charge of the international program and small modular reactor (SMR) deployment. He had the opportunity to go to Chalk River to be a vice president on the business development team. Half of Chalk River’s work in science and technology is directly for the federal government and the other half of their work is commercial. Most of the work historically has been supporting the CANDU fleet with life extensions through CANDU owners. CANDU reactors are heavy water moderated reactors that use natural uranium and have online fueling. One of the life limiting components are the pressure tubes. Chalk River does first tests of the fuel and pressure tubes under different conditions and provide the information to the regulator. Once the CANDU is at the end of its life, it is not shut down and decommissioned, but it can be refurbished. Refurbishment involves replacing the innards of the reactor, but the rest of the infrastructure can remain in place. The cost of refurbishment is usually a couple billion dollars. Large scale refurbishment is ongoing at Darlington which included a mock-up that workers can practice on. This allows the reactor to operate for another 30 years. Chalk River is the center for reactor sustainability and is the only laboratory in the world that supports life extension for the CANDU (heavy water), light water, and advanced reactors. The Ontario government committed to spending $26 billion refurbishing CANDU’s. Canada is one of the biggest new build markets and has the most robust supply chain and most supportive government environment of any place in the world.
6 - Nuclear Innovation in Canada
Bret Kugelmass: What are the things that slowed down progress in Canada’s nuclear industry?
Corey McDaniel: The stars are aligned for Canada because the country has the supply chain, supportive government, national laboratory to work out innovation, and an interest by more than two dozen small modular reactor (SMR) companies preparing to deploy technologies. Fuel qualification takes the longest, which Canada specializes in. NuScale’s philosophy was to use PWR (pressurized water reactor) fuel off the shelf. Regulator decisions regarding other fuels looked at for advanced reactors are to be determined. Canada has a progressive, risk-based regulator who has expressed a willingness to engage the vendors and have good discussions on how to deal with issues in reactors never seen before. Chalk River can help vendors put the fuel together for a demonstration or first-of-a-kind reactor. Advanced manufacturing and 3D fuel could have a lot of benefits and risks in the future. Non-proliferation is always a concern. Chalk River supports the government in nuclear safety and security on the forensics side.
7 - Nuclear Energy’s Keys to Success
Bret Kugelmass: What are some of the critical things that need to happen worldwide to put nuclear in the best position for an energy revolution?
Corey McDaniel: Corey McDaniel always analyzes problems from multiple angles. Nuclear cannot be solved purely from a policy side, otherwise, the Energy Policy Act would have led to a nuclear renaissance. It cannot be solved purely from a technology standpoint, otherwise, the passively safe AP-1000 reactors would be built all over the world. It cannot be solved purely economically, because nuclear has to be competitive in the market. New energy is clean energy. India, China, or developing countries would rather have any power instead of worrying about greenhouse gases. In the near term, the types of technologies looking to be deployed now will be in niche markets, such as cold, remote places where it is very expensive to deliver power. Longer term, humanity is heading toward more power that increases the quality of life for everybody and nuclear has a role to play. Markets cannot be forced if it doesn’t make economic sense. Eventually people will choose nuclear for it being cleaner energy.
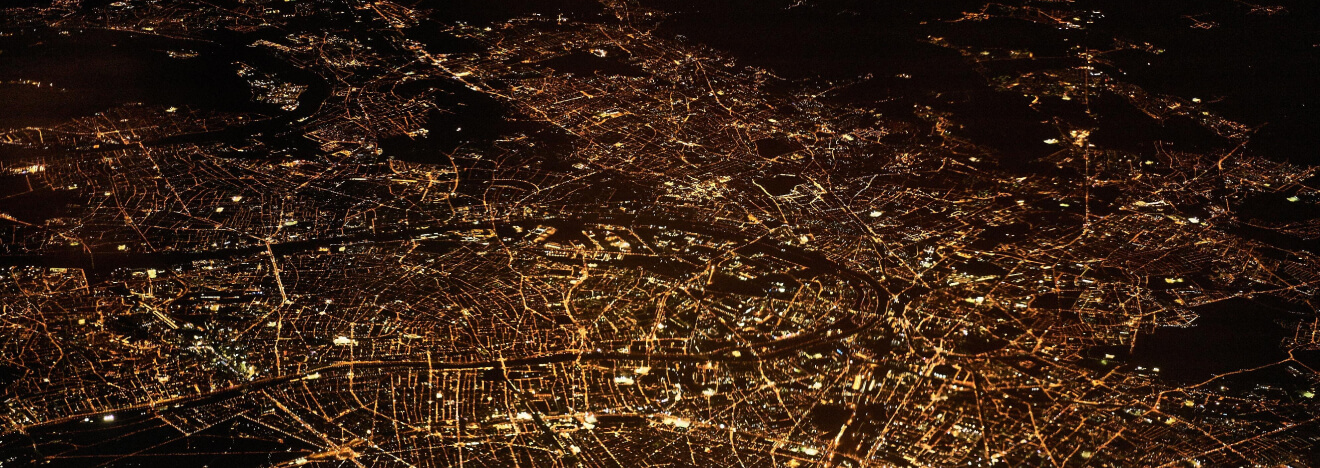
Sign up for our newsletter
No results found
Please try different keywords