TITANS OF NUCLEAR
A podcast featuring interviews with experts across technology, industry, economics, policy and more.
Latest Episode

1) The beginning of Brian’s career and his time in the United States Navy as a diver, as well as what drew him to engineering and nuclear
2) Brian’s initial journey to Oregon State and all of the research projects he’s had a hand in since then
3) Fostering a passion for nuclear in the next generation of nuclear engineers and why the researchers as just as important as the research itself
4) What challenges and successes the nuclear industry will face in the coming years and how to form your individual opinion on nuclear energy
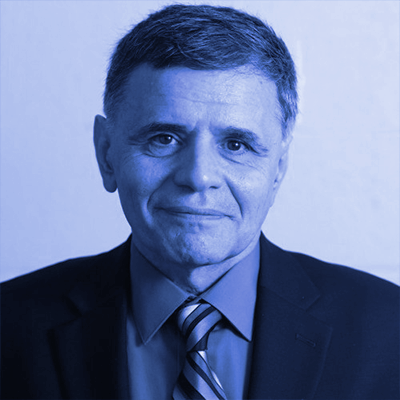
1) The Russian VVER and BN-800 designs
2) Developing optimization methods for the VVER at Penn State
3) How a lack of scientific support in Bulgaria caused nuclear scientists to move to western countries
4) Developing benchmarks with high fidelity simulations
Studying nuclear engineering in Moscow and Bulgaria (0:55)
0:55-7:02 (Kostadin describes his journey into nuclear engineering.)
Q. How did you get involved in the nuclear space?
A. Kostadin Ivanov is originally from Bulgaria. After high school, Kostadin recognized that he was skilled in physics and math. At that time, Bulgaria was beginning to build their first nuclear power plant: Kozloduy. Kostadin decided to study nuclear engineering, and attended a university in Moscow, where he received both a bachelors and master’s of engineering. Because the government of Bulgaria financed his studies, Kostadin was required to work at Kozloduy for three years after finishing his education. He began as an operator and then became chief engineer of one of the units. He then moved to the core design division. After, Kostadin applied for a PhD to study reactor physics at the Bulgaria Academy of Sciences. During this time, he worked at the Institute of Nuclear Energy, which is part of the Bulgaria Academy of Sciences. Kostadin stayed at the Institute after completing his PhD as a research scientist. He then applied for a Fulbright fellowship for optimizing core design and reducing the fluence on the reactor vessel, which is the damage caused over time from neutrons.
VVER designs (7:03)
7:03-15:45 (Kostadin discusses the VVER projects that he worked on when a PhD student and research scientist in Bulgaria.)
Q. Why don’t they put a sacrificial material in front of the vessel?
A. They did, but Kostadin performed calculations of how the neutron damage impacts the welds and the overall lifetime of the pressure vessel. The weld is affected more severely than other parts of the vessel due to the filler material used in the weld. The neutrons leave physical marks in the metal of the weld.
Kostadin worked on two major simultaneous projects after finishing his PhD. One project involved analyzing the new VVER-1000 reactors, which were more complex than the VVER-440. The 440 design was different from the US pressurized water reactors (PWRs) because the 440 had shroud, which changes calculation methods from the PWRs. The shroud absorbs neutrons and is similar to boiling water reactors where the assemblies are physically separated. The 1000 design adopted an open configuration, creating a more powerful reactor.
In most cases Russian reactor designs follow US designs, but in some cases Russia has introduced their own designs. For example, one of the new VVER-1000 designs changed to an 18 month cycle, which is similar to some of the US designs. These changes make the VVER more competitive. Some reactors in Finland have combined Western control instrumentation with the Russian VVER core design. There is also a trend to move to digital controls. However, the requirements for redundancy complicate the adoption of digital technology.
The BN-800 fast reactor (15:46)
15:46-19:33 (Kostadin explains his involvement with the BN-800 fast reactor project. He also discusses the BN-800’s configuration.)
Q. What does the configuration of the BN-800 look like?
A. In addition to the VVER project, Kostadin worked on the fast reactors. At the time, the Obninsk nuclear power plant near Moscow had the largest fast reactor center. The Bulgarian team was responsible for the optimization of core design, specifically looking at axle blankets. BN-800 is a fast reactor in Siberia that is currently in operation and produces 800 megawatts.
It is a hexagonal geometry with ceramic pellets on the inside. It has a sodium core reactor. It has both radial and axle blankets. The blankets contribute to reflecting neutrons but their main function is to produce plutonium from uranium-238. The blankets are assemblies of mostly uranium-238 which are breeding and are placed in rings. The rings are then reprocessed.
Penn State’s nuclear research projects (19:34)
19:34-24:44 (Kostadin describes the work he did at Penn State.)
Q. What kind of projects did you work on at Penn State?
A. After this project, Kostadin was working on a project with the International Atomic Energy Agency on fuel management. One of the people working on the project was Dr. Sam Levine from Penn State. Kostadin wrote Levine’s name on his Fulbright application as the person he wanted to work with. After 6 months, Kostadin was informed that he was one of ten people chosen to receive the Fulbright fellowship and would be working at Penn State. In 1993, Kostadin came to the US to work with Dr. Levine.
The project’s focus was developing optimization methods for VVER under Dr. Levine’s guidance. Kostadin also became involved with other projects in the department. At the time, the department was one of the strongest for nuclear research in the world, having strong connections with plants such as Three Mile Island and a total of 8 reactors in the state. Westinghouse and the Nuclear Regulation Committee (NRC) were also closely connected to Penn State. Kostadin worked with Westinghouse on a project involving the AP600 reactor.
Kostadin returned to Bulgaria after the fellowship, but immediately secured another fellowship with the International Atomic Energy Agency in Germany looking at the safety of VVER reactors. After eight months, Penn State reached out to Kostadin and asked him to return as a postdoctoral scholar. He eventually became a fixed research professor and resigned from the Institute in Bulgaria to stay in the US.
Deciding to stay in the US (24:45)
24:45-39:43 (Kostadin explains why he chose to stay in the US rather than work in Bulgaria.)
Q. What made you want to stay?
A. Kostadin saw a larger opportunity to develop professionally while staying in the US. Bulgaria was going through some rough political and social changes during this time. These changes did not encourage the scientific community in Bulgaria to flourish, and many members of the Institute left to work in western countries. The fall of the Soviet Union and the transition to an independent Bulgaria was peaceful, but the prior communist party was still powerful and guided decisions. Economic power and industrial growth became more important than science and education.
Kostadin stayed in the US and became an assistant professor. He then became an associate professor with tenure track in reactor design and safety analysis. After seven years, Kostadin became a distinguished professor. He focuses on using experimental data to validate computational tools. Kostadin began with reactor physics, but moved towards researching coupling with other physics to understand safety and design. He focuses primarily on the reactor core rather than the entire system.
Developing benchmarks (29:44)
29:44-42:00 (Kostadin explains his involvement in developing benchmarks. He also states the importance of developing high fidelity simulations to help industry address challenges.)
Q. How are benchmarks used to design reactors?
A. In addition to their connection with the NRC and the US industry, Penn State was also involved in international projects. Kostadin worked with the Nuclear Energy Agency OECD to develop the moot physics benchmark. The moot physics benchmarks were designed to test the moot physics tools and how they predict initial steady state and transient evolution. These benchmarks were developed using real reactors, starting with Three Mile Island. Many organizations from about 20 countries participated in the development and the NRC eventually adopted the benchmarks.
The main steam line and large break loca have different benchmarks. The moot physics is lost in large break loca. The main steam line break has a high probability to happen and has coupling effects. The main steam line break happens in one of the steam generators when the barrier between the primary and secondary loops is compromised. This causes overcooling in one part of the core, creating power gradients. Pressurized water reactors have two potentially dangerous situations involving mixing: temperature and boron mixing. Boron mixing causes a power spike. Kostadin’s computational models predict these accidents and the core response. Most of the feedback interactions happen within the core. Reactor physics, fuel, neutron behavior, temperature and coolant interact and Kostadin simulates these interactions and benchmarks.
It is challenging to model accidents because there are many parameters, especially on the local level. This means the team must approximate, introducing errors to calculations. They are moving towards high fidelity tools, though, that model everything on a higher resolution to see direct responses. Kostadin is now a chair in the working party of reactor systems with the nuclear science committee at the Nuclear Energy Agency. He is involved in several expert groups: uncertainty analysis in modeling and moot physics validation. He now works on creating more sophisticated benchmarks. They use the Consortium of Advanced Simulation for Light Water Reactors (CASL) to validate the new high fidelity systems and create new international benchmarks. Understanding what is happening on the pin level versus the assembly level avoids approximations and reduces error. With better predictions, the margin is known and can increase operational flexibility. Crud, or the build up on the outside of fuel pins, is also better understood with high fidelity modeling. These simulations helps address industry challenges.
Working with the government to secure the future of nuclear (42:01)
42:01-46:06 (Kostadin outlines the challenges that the industry faces and how working with the government can help secure the future of the industry in the US.)
Q. Where do you think the industry will go in the future?
A. Kostadin believes that the industry has challenges. The safety issues of Fukushima have been addressed and Kastadin believes there are technical solutions to other challenges that the industry faces. We now need to find political solutions to challenges. Cost and time are the major challenges that must be overcome to be competitive with other energy sources. However, Kostadin sees a positive outlook for nuclear in the US because of the new advanced reactor developments and the support of the government. North Carolina State University has formed an alliance with the state government to work with the nuclear sector and the Carolinas have extended the life of the existing plants. Kostadin believes the nuclear industry must be proactive to ensure its future.
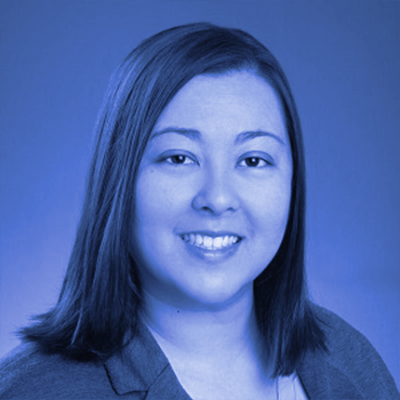
1) How radiation changes materials within a reactor
2) The advancement of electron-beam welding and the potential to decrease cost and extend reactor lifetimes
3) Strengthening materials by precisely deforming crystal structures with radiation
4) The need to deploy technological advancements to move the nuclear industry forward
Becoming interested in materials (0:16)
0:16-6:33 (Janelle explains how she ended up in the nuclear industry, discussing how her interest in materials arose.)
Q. Can you tell us about growing up in Hawaii?
A. Janelle Wharry grew up in Hawaii and had always wanted to enter science and engineering. As a high school student, she attended a camp for prospective engineers at the University of Illinois. This is where she was first introduced to nuclear, motivating Janelle to apply to universities with nuclear engineering programs for university. After completing her undergraduate degree at Michigan State, Janelle entered the power industry, working at Duke Energy in core design. There, she realized that unnecessary plant shutdowns were due to materials issues. Fuel pin leakages, for example, are material issues that can cause a shutdown. This is when the coolant water comes into contact with the fuel pellets in the fuel pin, releasing fission gases into the rest of the coolant water. Janelle also looked into fuel cladding to understand the effects of different types of materials on overall reactivity. Cladding is typically zirconium based alloy and Janelle explored alternative alloy options. These material challenges sparked Janelle’s interest and she returned to school to study the effects of radiation on materials during her PhD. She is now a professor of nuclear engineering at Purdue University.
How radiation changes materials (6:34)
6:34-11:37 (Janelle explains the impacts of radiation on materials.)
Q. What are the effects of radiation on materials?
A. Most materials in a reactor are metallic or ceramic. The materials are made of atoms arranged in a lattice. Neutron radiation knocks atoms out of their lattice position. Gamma radiation does not knock atoms out of place but some electronics can. When atoms are moved, defects are formed. Some small defects will clump together to form larger defects, causing voids. These voids are pockets of vacuum, which cause materials to swell. Other atoms reposition around these voids, creating a more stable structure. Materials research is focused on intelligently designing materials to take advantage of this to create new materials that are more resistant to radiation. The fuel, clad and steel are most subject to radiation damage.
Material lifetimes (11:38)
11:38-17:51 (Janelle discusses the lifetime of materials and how welding pieces of a pressure vessel rather than forging can extend the overall lifetime.)
Q. How long are the lifetimes of the different materials?
A. Cladding and fuels are replaced every few cycles. Fuel assemblies are usually replaced every three fuel cycles, which are typically 18 to 24 months long each. These materials only need to last for about 6 years. Pressure vessels are not replaced and are therefore more critical when it comes to resisting radiation damage. These materials are not replaced because they are forged as a single piece, which has a high cost associated with it. Janelle believes figuring out a way to build the vessel in multiple pieces, allowing for the more damaged parts to be replaced, would greatly extend the lifetime of a reactor. Radiation exposure causes the vessel to become brittle over time, meaning it can absorb less mechanical stress. On an atomic level, the brittleness in pressure vessel steel is caused when different chemical species cluster together. The alloy begins with some other elements within it, such as copper, silicone, nickel and manganese. During radiation, these elements cluster together. Dislocation loops also form, which are faults within the material, causing the material to become brittle. Annealing will get rid of these dislocation loops, greatly decreasing embrittlement and increasing the lifespan of the reactor vessel. The problem arises when attempting to anneal the entire 20 foot structure, but building the core in multiple pieces would enable easier annealing.
Welding versus forging (17:52)
17:52-28:18 (Janelle explains how welding is cheaper than forging and how the new electron-beam welding method is on the path to NRC acceptance.)
Q. What is the push back on welding rather than forging?
A. Janelle notes that forging is what has always been done but believes welding pieces on site would be easier, faster and cheaper. Utilities must present new ideas in the US to the Electric Power Research Institute (EPRI), which represents all US utilities. EPRI has been exploring welding rather than forging pressure vessels using electron-beam welding, which does not use a filler metal and is low heat. This new welding method creates welds that look no different than if the vessel had been forged. Janelle is currently working on radiating these materials to produce fracture tests to understand the embrittlement of the material. She will then report her findings to show that this new welding method can produce vessels that can withstand radiation. After these tests, Janelle hopes to move the material through the qualification process to make sure it follows pressure vessel codes and meets all specifications. The American Society of Mechanical Engineers (ASME) will then write the electron-beam welding method into code, which the Nuclear Regulatory Committee (NRC) can then reference. This would set the industry on a path to the NRC accepting this technology for reactor use.
Improving materials with radiation (28:19)
28:19-35:08 (Janelle discusses the various projects she is working on, primarily looking into how irradiation can be used to induce positive defects to improve materials.)
Q. What else do you work on?
A. Janelle works on many “cook and look” projects where an object is irradiated and they see what happens. Many tests are carried out at the Advanced Test Reactor at Idaho National Labs. Janelle also works in the tailoring of mechanical and material properties using irradiation. Janelle believes this is a future path for nuclear science. Instead of thinking about radiation mitigation, Janelle is interested in exploring if radiation causes positive effects that scientists can take advantage of to improve materials. Janelle is looking at lithium ion batteries for energy storage. Defects in the metal oxide within a battery can cause more lithium to enter the metal oxide, increasing the energy storage capacity of the battery. Janelle is exploring if radiation can be used to induce these positive defects. She is also researching how to use irradiation to build specific features into metals to influence how it will respond to mechanical stress.
Strengthening materials with deformation (35:09)
35:09-44:16 (Janelle explains the different crystal structures found in materials and how irradiation can be used to change these structures, strengthening the material.)
Q. What are the different deformation modes?
A. Localized deformation, or twinning, occurs in metals at low temperatures. This is when only localized regions are affected. Face transformation occurs under high pressure zones and is when the crystal structure transforms into another crystal structure. This could potentially increase ductility, strength or toughness of a material. The primary crystal structures of face-centered cubic and body-centered cubic. Face-centered cubic is a typical crystal structure for steels and is when all atoms are arranged in a cube. Body-centered cubic structure is similar to the face-centered cubic structure, but has one atom in the center of the cube. A material with different crystal structures tends to be stronger. It is possible that we will be able to irradiate materials to precisely change structures someday. We can change the energy level and particle type used in irradiation to induce different microstructure changes at different depths within the material.
Deploying technological advancements to move the industry forward (44:17)
44:17-45:23 (Janelle discusses her view of the industry ten years from now. She calls for significant innovation and for the industry to deploy these advancements.)
Q. Where do you think the industry will be 10 years from now?
A. Janelle sees the need for significant innovation. She also calls for the industry to harness and deploy the technological advancements made in reactor designs. Janelle believes this will transform the way the industry works in the US, influencing changes in other countries.
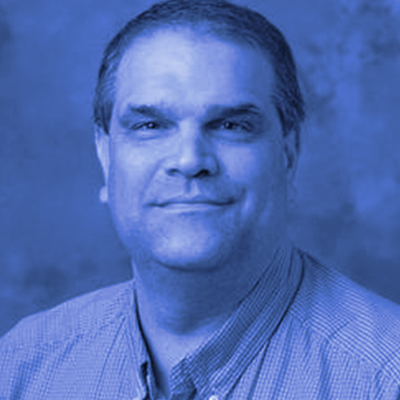
1) Robert Bean explains how he first became interested in nuclear engineering and what sparked his interest in academia
2) Robert’s post-graduate path to becoming an expert in radiation detection and how he became involved in designing nuclear facilities for safeguards
3) Robert reflects on his time in Washington as an advisor to the NNSA and how it brought him back to Purdue University as a full-time faculty member
4) A highlight of the Purdue University reactor and some of the current research projects utilizing the facility, including projects in physics and health science
5) Robert Bean explains some of the challenges of nuclear reactor deployment and potential benefits of building small modular reactors
Early experience in nuclear academia (1:22)
1:22-10:51 (Robert Bean explains how he first became interested in nuclear engineering and what sparked his interest in academia)
Q: How did you get into the nuclear space?
A: Robert Bean originally wanted to go into the Navy to become a fighter pilot. When he found out he was too tall to become a pilot, Robert decided to pursue engineering at Purdue University so he could design airplanes. He was also interested in nuclear, which led him to fusion-powered spacecraft. Robert didn’t end up working in that field, but it got him into the nuclear space. Between freshman and sophomore year, Robert spent two years as a missionary. When Robert returned to school, he found that the nuclear classes really clicked and were the most interesting part of his education. Nuclear is abstract because you never see the thing you’re working with.
Robert sees his students gain confidence as they go to their first job and show that they do know all the things they’ve been talking about in school. At the same time, Robert hopes his students realize they don’t know everything and strives to provide that balance in his classes. The primary classes Robert teaches are the radiation detection classes. One of the labs is the neutron demonstration, which allows students to give predictions, complete the experiment, and figure out why they were wrong.
Becoming a radiation detection specialist (10:51)
10:51-24:35 (Robert’s post-graduate path to becoming an expert in radiation detection and how he became involved in designing nuclear facilities for safeguards)
Q: What was your first professional job outside of school?
A: Robert Bean stayed in college for his PhD, which later allowed him to become a professor, and paid for graduate school by being an employee of the university and teaching radiation detection classes. His first job was at the Idaho National Laboratory as the radiation detection person for the safeguards team. Safety is what’s done to make sure people don’t get hurt, equipment doesn’t get destroyed, and the public doesn’t get exposed to the radioactive material. Safeguards are things done to make sure the radioactive material is not lost or stolen. A significant fraction of safeguard work is done by measuring the radiation given off by the nuclear material. Robert’s first job was to teach the technicians on the team how to use the radiation detection equipment.
All radiation interaction leads to ionization and excitation of electrons. The detector collects a signal to provide knowledge something with radiation interacted with the detector. Every radioactive isotope gives off a unique signature of gamma energy and quantity. This spectrum can be measured to identify what the radioactive source is and how much of it there is. Typically the gamma rays emitted by radioactive decay are measured. Since uranium-235 and uranium-238 have different half-lives, gamma rays from each can be distinguished and the enrichment can be determined by looking at both. An Active Well Coincidence Counter injects a neutron source so the neutrons can cause fission. This active interrogation allows one to look at the neutrons produced from the fission. This is helpful when gamma rays cannot be used in measurement due to shielding. Plutonium almost always has some measure of plutonium-240, since plutonium-239 absorbs a neutron but doesn’t fission since it is in neutron flux. Since plutonium gives off neutrons, neutrons don’t have to be injected to take a measurement.
Robert Bean worked in radiation detection at Idaho National Labs for 10 years. He eventually shifted over from the safeguards team in the field into more international safeguards and safeguard design. He looked at how to change a design process for a facility so it is designed with the safeguards in mind from the beginning. Most of the benefit comes from getting the right people involved at the front end and making sure the safeguards inspector can get access to the right areas to get the information needed.
Teaching others about nuclear energy (24:35)
24:35-38:11 (Robert reflects on his time in Washington as an advisor to the NNSA and how it brought him back to Purdue University as a full-time faculty member)
Q: What happened next in your story?
A: Robert Bean had always considered that if he left the lab, he would return to university. At the time he accepted an offer to Purdue University, Robert was on assignment in Washington, D.C. as an advisor to one of the NNSA (National Nuclear Security Administration) groups that funded a lot of the projects at Idaho National Labs. The program aimed to bring the lab prospective to Washington and to bring the Washington perspective back to the lab. Once the assignment in Washington ended, Robert put in his notice at Idaho National Lab and started working at Purdue. He is now taking care of the reactor and teaching the same labs he did as a graduate student, but now as part of the faculty. Robert has acquired special knowledge in the specific area of radiation that allows him to bring his specialization to the team.
Robert does a lot of public tours of the reactor, which provides him with opportunities to teach people who know nothing about nuclear a little bit about the technology. He utilizes and promotes the analogy of cooking something on the stove. Not many people, other than the mechanical engineers, understand the heat transfer and the chemistry in cooking. However, every person knows how to use the stove properly and how to notice when something is wrong. The public doesn’t have to understand the nuclear physics; they have to understand whether the plant down the street is operating safely.
Some things that can be measured lead to associated standards, such as lead, arsenic, or radiation. When the ability to measure gets better, standards are dropped to make it safer. However, wisdom must be used to figure out when to do that. One motto at Idaho National Lab is, “Don’t let perfect be the enemy of good enough.” When “good enough” is achieved, one should question whether they should do more. Sometimes the answer is yes, sometimes the answer is no. A real diverse team needs diversity of experience, ways of thinking, and ways of approaching a problem.
Purdue University reactor research (38:11)
38:11-46:04 (A highlight of the Purdue University reactor and some of the current research projects utilizing the facility, including projects in physics and health science)
Q: Tell us about the reactor at Purdue University.
A: Purdue University has the first all-digital reactor under Nuclear Regulatory Commissioning (NRC) licensing. Critical is a state of the reactor where the neutron balance is maintained and is absolutely not related to how many neutrons per second are currently flying around in the reactor. A reactor could be critical at low power or high power. In theory, it could be critical zero power because the reactor is in a state in which the chain reaction would be maintained but there are currently no neutrons present. The reactor is licensed for 10,000 W of thermal heat which is in an appropriate range for providing neutrons for some irradiation for people and seeing temperature effects. Because of the small size, the potential for accidents is basically nothing, which allows students to be on the controls with an operator standing by. The fuel is a two foot cube and includes 16 fuel assemblies with a row of graphite reflector assemblies. It sits at the bottom of a 20 foot deep pool.
Neutron activation analysis is the exposure of something to neutrons and using a gamma detector to detect and measure radioactive isotopes. One physics professor at Purdue is looking at variation in half-life, suspecting that physics is a factor and that the variation is not just because of experimental uncertainty. His experiment is to put down different shapes, like a sphere, cylinder, or cube, of material into the reactor to be exposed to neutrons and measure any differences. A health sciences professor at Purdue has developed a technique to measure heavy metal poisoning. China is having a lot of problems with mine tailings contaminating water supply as it goes through industrialization. She developed a technique which puts a radiation source on someone’s shin bone and uses an x-ray detector to see if there is heavy metal inside the bone. The possible, small but non-zero, risk from the radiation is balanced with the fact that the person might have heavy metal poisoning. If there is heavy metal contamination in someone’s body, it will also be in their toenails. So the professor took some toenail clippings and irradiated them in the reactor. Some of the atoms absorbed neutrons, were put on the gamma detector, which showed that heavy metals were present. She is now working to demonstrate the relationship between the heavy metal content of the body and the heavy metal content of toenail clippings.
Future of small modular reactors (46:04)
46:04-56:20 (Robert Bean explains some of the challenges of nuclear reactor deployment and potential benefits of building small modular reactors)
Q: Why can’t we put one of your reactors in every town?
A: The Purdue reactor is a big open tank that doesn’t produce any electricity. One the reactor starts to be scaled up, the complexity grows dramatically. There is a breakpoint in the economics whether it would be worth building. However, a large number of small reactors could be built versus the small number of large reactors that currently exist. The licensing process is working on small modular reactors (SMR), which serve two primary goals. One goal is getting nuclear power to places like Indonesia that doesn’t need 1,000 MW plants, but instead needs lots of little reactors on their islands. The first SMR to get hopefully deployed will be NuScale, who is working with the Department of Energy to build a prototype at Idaho National Lab.
The Purdue reactor is allowed to make 10,000 W of thermal energy, but this would not be worth it economically outside the university environment because of the loss. In order to take the reactor design and scale it to 1 MW, the reactor would need more cooling power and piping, but is not much more complex. The dream of nuclear power is to produce about 100 MW, which is enough for a city and can still be built small. Once one reactor is built, it creates a revenue stream through electricity production, or possibly district heat, that could fund more reactors. If process heat is the only product, the complexities become a lot less restrictive. Industry has shown an interest in nuclear process heat. Even though they aren’t prepared to take the risk in producing it, they see the benefit if it was to get developed.
Robert Bean imagines that the future of energy in 100 years is probably orbital solar stations. The problem with solar is that the sun only shines half the time on your system. In the time to reach that point, a nuclear system of some kind will be needed. Nuclear offers a lot of advantages, but it does have a high capital cost. Once these facilities are built, they have decades-long lifetimes and have the cheapest electricity production, with the exception of natural gas in the current economy. The technical, social, political, emotional, and economic sides of nuclear cannot be separated. There will continue to be an energy mix, but as long as nuclear maintains its safe and reliable track record, it will continue being part of the mix.
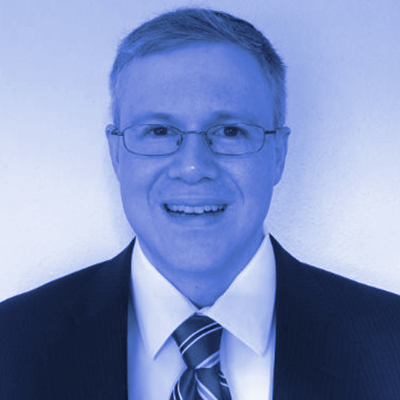
1) Allen’s early work in prevention of mutual interference in the Naval Reactors submarine force
2) How Allen transitioned from being a Naval Submarine Officer to a biomedical graduate student studying modification of biological cells
3) How Allen’s research on electromagnetic interference at GE has been applied to create a non-linear transmission line at Purdue
4) Impacts on nanostructures caused by intersections of different mechanisms in electron emissions and gas breakdown
Allen’s intro to the Naval Reactors Program (0:26)
0:26-11:29 (Allen discusses his introduction to nuclear power in the Naval Reactors Program)
Q: Tell me about your nuclear start in the Navy.
A: Allen Garner got his start with nuclear while in the Navy and still serves in the Navy Reserves. This is a part-time role that he juggles with his role as a professor at Purdue University. Allen starting doing submarine work in the Navy, focusing on prevention of mutual interference. He studied nuclear engineering at the University of Illinois as a Naval ROTC student, eventually placed in the submarine force by the Naval Reactors. The Naval Reactors Program is responsible for managing and administering the Naval nuclear propulsion program, including submarines, aircraft carriers, and research and development of Naval nuclear propulsion.
After Allen went through Naval Nuclear Power School, he completed Prototype, where he learned nuclear hands-on, and Submarine Officer Basic Course, where he learned the basics of the submarines. He then went on to his Junior Officer tour on the USS Pasadena as the reactor controls assistant and chemistry and radiological controls assistant. During Allen’s tour, the submarine went through an analog-to-digital instrumentation change out. The Navy has a few remaining prototype areas which uses moored training ships that are brought into port to train operators. During training, operators go through many different ways to cover the reactor in emergency situations.
Post-graduate biomedical research (11:29)
11:29-22:23 (Allen reviews his post-graduate research in bioelectrics at Old Dominion University and in nuclear engineering at the University of Michigan)
Q: Where did you go after the Navy?
A: After ROTC during his undergraduate studies, Allen Garner took a leave of absence from the Navy to get his first Master’s degree at the University of Michigan. Allen did theoretical studies of crossed-field devices, which are used in RADAR systems in the Navy and the Air Force. A crossed-field device has a parallel plate with voltage in the middle, creating an electric field. The magnetic field is perpendicular to the plates, which turns the electrons. Towards the end of his Junior Officer tour, Allen put in for the Navy to send him to shore duty and was sent to Norfolk, Virginia. He did bioelectric research at Old Dominion University (ODU), which uses intense non-ionizing radiation to modify biological cells. When a voltage is applied, the water in the membrane moves and pushes proteins and lipids out of the way. This creates holes which, if big enough, all everything to leak out and the cell to die. If the size of the holes is controlled, things can be inserted and the hole can reseal.
Allen’s advisor at ODU worked on intracellular manipulation. Inside the cell, mitochondria play a critical role in regulating apoptosis. If the cells aren’t dying properly and don’t undergo apoptosis, cancer can develop. Nanopulses can be used to charge mitochondria and allow them to leak enzymes that initiate the apoptotic pathway. The apoptotic pathway is massive and extremely complicated.
After finishing up at Old Dominion, Allen returned to the University of Michigan to work on his PhD in nuclear engineering. One topic he studied was modeling tumor growth. Tumors are very evolved structures which work symbiotically with the body, growing by diffusion and bringing in blood vessels to continue to feed them. The recruitment of the vasculature, or mastocytosis, makes certain tumors spread. Allen loved biology in high school and considered majoring in genetic engineering, which he picked up at ODU and in his PhD program before going to work for GE.
Electromagnetic physics research at GE (22:23)
22:23-40:10 (Allen explains his research at GE in electromagnetic interference how he applied it non-linear transmission line research at Purdue)
Q: What did you do at GE?
A: Allen Garner was originally hired into GE as a biomedical engineer, which morphed over time into an electrical engineering position, and eventually an electromagnetic physicists. He was brought on to work on a project involving microscopy. Allen got involved in radiation detection instrumentation and non-proliferation early on, later working on satellite visualization in terms of resolution calculations. During his last five years at GE, Allen spent most of his time modeling thermal stresses on flip switches for satellites. The RF lab would make very small chips to be used on the satellites, but the temperatures in space were much different than on Earth. Shrinkage happens due to the temperature coefficient which creates forces that are strong on sharp angles. Allen used finite element analysis to calculate where the strongest forces were.
Another one of Allen’s projects at GE was focused on electromagnetic interference. It was originally funded by GE Plastics, who was looking to increase their market share. Plastic is a dielectric, but it can’t be used to shield electromagnetic radiation. Things are going to become more and more electric and electromagnetic interference becomes more of a concern. The question was whether plastic can be made more shielding by making it look more like a metal. Percolation is when a composite material and filled with a filler material to make it look like the metal material. The aspect ratio of the material determines the percentage volume loading of filler material to make it look like a metal. Finite difference time domain is important in electromagnetic radiation because things vary with change. Allen hit upon a semi empirical method that allowed him to develop fitting parameters that would be predictive. Experiments with stainless steel in different aspect ratios were completed and results fed into the predictive model.
Purdue University has funding from the Office of Naval Research to take these concepts and apply it to a non-linear transmission line. A transmission line is a cable with dielectrics represented by a combination of capacitors and inductors. If the capacitance is changed to be a function of voltage and/or the inductor varies with current, a non-linear transmission line is created. The non-linearity creates an electric shockwave which generates a radiofrequency signal. This can then be used to drive a high power microwave system to project a radar or a pulse.
Current Projects at Purdue (40:10)
40:10-49:16 (Allen summarizes current, ongoing research projects at Purdue related to electron emissions, gas breakdown, and nanoelectronics)
Q: What’s another project you’re working on at Purdue?
A: Allen Garner has two other projects at Purdue, one funded by the Air Force and one funded by the Navy, which look at electron emission and gas breakdown. A plasma has ions going around and voltage is applied to cause electrons to move. As electrons are stripped, they interact with the neutral particles which adds on to the standard secondary emission that helps to drive Paschen’s Law. Allen’s group has analytically derived equations that show how the breakdown voltage varies linearly as the gap distance is reduced. Practical applications include microsatellites, propulsion and combustion, and biomedical applications. The angle of the electrons creates field enhancement. One experiment shows where the transitions between the emissions regime, which has never been looked at before. Another student derived a triple point, or a nexus, which is an intersection of different mechanisms. This student put in collisions, standard space charge limited emission, and field emission, showing where all three mechanisms intersect. The nexus between thermionic emission, space charge limited emission, and field emission was also looked at. Understanding how these mechanisms interplay assists in design. As devices are made smaller and smaller, an important question is related to the impact of heat.
Some of Purdue’s projects on the bio side are potentially very interesting. One looks at antibiotic-resistant microorganisms and using electric pulses with drugs to treat them. On the non-bio side, there are a lot of applications in nanostructures and nanoelectronics, such as how 5G waves interact with the structures.
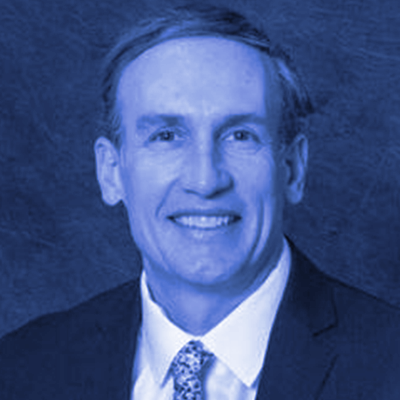
1) Jason Harris reflects on how his early interest in biology led to a career in nuclear engineering
2) Jason leans into health physics and how radionuclides are distributed throughout the environment
3) A comprehensive look at nuclear power plant emissions and the birth of the nuclear security field
4) Methods of analyzing and assessing security culture at organizations and how it impacts risk at a nuclear facility
Biology and Nuclear Engineering (0:31)
0:31-9:03 (Jason Harris reflects on how his early interest in biology led to a career in nuclear engineering)
Q: Where did you grow up?
A: Jason Harris grew up in Baltimore, Maryland. As the oldest of four from a blue collar family, Jason was the first in his family to go to college. Originally, he wanted to go to med school and started out studying biology and chemistry. Jason took a job in the environmental toxicity testing field after graduating and his wife-to-be took a job at an R&D start-up working in the clean energy sector. They needed scientists to work specifically on radioactive waste amelioration and tritium separation, so Jason was hired to work in the lab. It was this job that shifted Jason’s trajectory as he started to understand nuclear energy and nuclear waste. One of the consultants for the company was Dr. George Miley, a professor at the University of Illinois at Urbana-Champaign in the nuclear engineering department. He was the inspiration for Jason and his wife-to-be to pursue graduate school, both receiving their Master’s and PhD’s.
Tritium is radioactive hydrogen and is a product in the nuclear generation process, as a fission product and through other neutron activation reactions. There are three forms, or isotopes, of hydrogen: protium, or regular hydrogen; deuterium, which has a neutron; and tritium, which has two neutrons. Because hydrogen is such a small element, it is very hard to separate. The start-up Jason worked at developed a hollow-core fiber with a mesh inside, a semipermeable membrane. Tritiated water could be drawn through it and separated. Tritiated water and normal water also have different freezing points, so it could be separated that way, but it was very energy intensive.
Environmental Distribution of Radionuclides (9:03)
9:03-24:47 (Jason leans into health physics and how radionuclides are distributed throughout the environment)
Q: What did you focus your efforts on in grad school?
A: Jason Harris and his wife were brought into the University of Illinois for grad school by Dr. Miley. Dr. Miley’s area was fusion and low energy nuclear reactions (LENR). Jason worked for Dr. Miley at first, but realized his interests were tied to biology looking at the potential health effects from nuclear and went to do his Master’s with a different advisor in the health physics area. The linear no-threshold (LNT) model for radiation protection is still controversial because research has shown that it doesn’t hold in all situations. However, it has its place for regulatory purposes because it is a clean, easy-to-use model. If one looks at individual scenarios or situations, such as cancer development, it’s been shown that LNT is not the model in the initiation, promotion, and development of tumors. It has been very expensive for a lot of industries to follow the LNT model because the assumption is that any dose of radiation has an effect. The stance of the Nuclear Regulatory Commission (NRC) is that they don’t support any industry and don’t show any favoritism. There are some changes, like NRC’s process of going to a risk-informed approach, but it is not increasing the speed at which regulations are changed or new reactors are designed. There are still some debates and differences in the science on which models hold. There are conflicting studies that have shown some detriment at the cellular level that may or may not create an adaptive response. What’s keeping LNT around and alive is that there is still not a biomarker or mechanism for cancer development.
Jason is a health physicist, nuclear engineer, and biologist. There is risk versus benefit for everything related to radiation. In a lot of technologies in which radiation is emitted, there is some byproduct. Some radionuclides have long enough half-lives that they stick around in the environment, such as cesium-137. With a thirty year half-life, it will be down to a percent of what’s left after about seven half-lives, but is still in the environment for hundreds of years. After they are released, the way they distribute through the environment depends on their chemical form. Cesium bonds to different types of soils and clays, limiting uptake by people in most cases. Iodine is taken up readily by the thyroid gland, but it has a much shorter half-life. Radioactive waste is really a political problem. There are still fission products and transuranics that will last hundreds or thousands of years, but they are not the ones that should be worried about. Nuclear power operations dilute a waste stream that is deemed not harmful, which will have to be done at Fukushima to release the water.
Nuclear Power Plant Emissions (24:47)
24:47-39:23 (A comprehensive look at nuclear power plant emissions and the birth of the nuclear security field)
Q: Tell me about your research now and what you focus on.
A: Jason Harris did some work looking at the dose impact from nuclear power emissions, which is minimal. He partook in studies, over the course of a decade, looking at every nuclear power plant in the U.S. and what they release through gaseous, liquid, and waste effluence. Each power plant has a comprehensive monitoring program to make sure what they release is not accumulating in the environment and not creating harm to the environment or individuals. Jason also compared these results to some of the accidents like Chernobyl and Fukushima. People are focused on Fukushima and the reactor and what was released there. The earthquake and the flooding harmed and impacted the local industry and chemical plants. The releases on those sites were much worse in terms of health effects than Fukushima. There is still some fear and concerns people have with radiation, but Jason sees that changing. People are starting to accept the technology and see that it is safe.
Jason’s work has also taken him into the nuclear security area. Before he came back to Purdue as a professor, Jason was at Idaho State University and had a joint appointment with the Idaho National Lab. He was also the associate director for the Center for Advanced Energy Studies. Jason got a call out of the blue from someone at the International Atomic Energy Agency (IAEA) who was putting together an education program in nuclear security, which did not exist. After 9/11, there was a real concern about the possibility of using nuclear, radiological materials for malicious purposes like a terrorist attack. Four scenarios are considered for security against a substate actor: getting ahold of an intact nuclear weapon, getting enough nuclear material to build an improvised nuclear device, getting radiological material to make dirty bombs or radiological dispersal device, and sabotage. Jason’s group specializes in risk analysis and assessment for radiological security. They developed one of the most comprehensive models that looks at every possible parameter that determines risk at a radiological facility. This model looks at both physical parameters and human factors that could affect the facility’s security, such as weather or a power outage.
The Human Factor in Nuclear Security (39:23)
39:23-48:20 (Methods of analyzing and assessing security culture at organizations and how it impacts risk at a nuclear facility)
Q: How do you include the human factor in risk models?
A: It is very difficult to model human factor, so Jason Harris and his team focuses on assessment of the security culture at each organization. It’s hard to pinpoint on an individual, but looking at a facility as a whole, they can determine whether people are happy at work or whether workers are disgruntled. Another thing being developed is the use of algorithms in concert with sensors and AI to track individuals and detect very subtle differences in behavior, which has both safety and security applications. An algorithm could be developed to see if the reactor operator is doing things they shouldn’t be doing, by looking at their actions, behaviors, or devices they are manipulating that are not typical behavior. Nuclear is getting close to operating without operators, but there might be some scenarios in which have an experienced person to make a call is wanted.
Jason is optimistic about nuclear power in the U.S., even though plants are shutting down. The turn needs to happen soon, however, especially from an economic standpoint. A lot of people thought that regulations would kick in for natural gas and would help soften the blow in the difference in cost. Climate change is very important and people must get on that ship and steer it the right way very soon. Part of the problem with large scale nuclear is, not only cost, but the time it takes to build a new plant. There is hope for moving towards advanced nuclear like small modular reactors (SMR) and microreactors, but they need to be able to get through the regulation process quickly. Nuclear throughout the world is flourishing and people see nuclear as the energy of the future because of their own energy security needs and lack of resources.

1) How Three Mile Island sparked Juan’s interest in nuclear power and his journey to becoming a strategist for the Army’s Logistics Innovation Agency
2) Saving lives and combating climate change by improving Army logistics by adopting nuclear power
3) The current progress in developing a small modular mobile nuclear power plant prototype
4) The importance of electrifying the military to maintain the technological edge
Becoming Boom Boom Vitali (0:08)
0:08-11:11 (Juan explains his motivation to enter the nuclear industry and his journey to becoming a strategist for the US Army.)
Q. How did you first get interested in nuclear?
A. Three Mile Island first sparked Juan Vitali’s interest in nuclear. Juan was awarded a scholarship to the University of Florida where he studied nuclear engineering. At the time Venezuela, where Juan is from, was interested in building nuclear reactors to replace fuel exports and planned on becoming a nuclear power of Latin America.
At the University of Florida, Juan focused on power plant engineering. He then got a Master’s in radiation technologies and a PhD in plasma physics. After, Juan worked on molecular spectroscopy with a special focus on flame and combustion at the Air Force Research Laboratory. There, Juan helped find the solution for the halon problem, which is a strong ozone depleter. The Air Force F-16 tank uses halon 1301 as an explosion suppressant which is then dumped into the ozone. Juan’s desire to protect the environment became his underlying motivation to find an alternative to halon.
Juan was then recruited by the Office of the Secretary of Defense (OSD) as the Director of Operational Testing Evaluation of Live Fire Testing. His role was oversight of weapons systems and understanding how fire extinguishing systems work. This involved many test explosions, earning Juan the nickname “Boom Boom Vitali.” After three years at OSD, Juan became a faculty member at Georgia Tech Research Institute. He then entered the Army to test and evaluate aviation systems before transitioning to the Logistics Innovation Agency where he is now a strategist.
Nuclear’s role in Army logistics (11:12)
11:12-18:30 (Juan explains the importance of logistics and why nuclear can decrease casualties.)
Q. What is so important about logistics?
A. Logistics are needed to win wars. Logistics involves the movement of goods and people, doing maintenance, and providing fuel and supplies to battlefields. Fuel, water, food and ammunition, known as ordinance, are the primary goods transported during times of war to enable fights to be won. This supply must therefore be optimized to provide for the needs of war while avoiding becoming a burden. The need to improve logistics became apparent during the Iraq and Afghanistan wars when 50% of casualties occurred during fuel and water transportation.
In 2013, Congress saw the opportunity of modular reactor technology and decided to explore the idea of a modular reactor in a battlefield. With nuclear, synthesizing fuel in the field is a possibility. Producing fuel at a site through CO2 capture to create JP-8, a type of fuel similar to diesel, is a possibility. Fischer-Tropes Synthesis, which is a 50 year old patent, is the process of capturing CO2 to reconstitute hydrocarbons. The Navy already does this with seawater, however capturing CO2 from the air is more difficult. Using nuclear will allow the US to increase logistics efficiency thereby saving lives while also contributing to climate action.
First steps towards mobile nuclear plants (18:31)
18:31-27:35 (Juan describes the military’s process of beginning to explore mobile nuclear plants.)
Q. What is your process to begin thinking about how to bring mobile nuclear to the military?
A. The Defense Science Board came together after Congress began exploring the idea of modular reactors in the field. A report was issued that broadly laid out what the options, opportunities and challenges were and explained the issues that needed attention. The report also recommended the Army take the lead.
Juan’s team took on the research project. The Army used to have a reactor program which was established in the wake of Eisenhower’s Atoms for Peace. Juan’s team produced the Study on the Use of Mobile Nuclear Power Plants for Ground Operations report which laid out the challenges and opportunities of nuclear power. The opportunities focused on the favorable political environment, the readiness of technology, the desire to rebuild the US nuclear industry and the ability to make supply lines survivable to protect soldiers. The challenges included not having built a reactor in many years, no significant demand for enriched uranium and the overall challenge of restarting the nuclear fuel industry. Additionally, it is unclear what the international legal process is when a reactor is shipped across an ocean or flown over other countries.
Juan sees the opportunities to be far greater than these challenges. Operational casualties in nuclear are far less than other forms of energy production. Nuclear also allows for the elimination of entire supply chains. Despite this, the Army has yet to make a decision on the topic.
The electrification of the Force (27:36)
27:36-37:59 (Juan explains how adopting nuclear will enable the military to become electrified, maintaining a technological edge. He describes the current progress in designing a small modular mobile reactor and the measures in place to protect the reactor if under attack.)
Q. The US Army seems like it is in the best position to take on a nuclear research project, right?
A. The other reason for adopting nuclear is to move towards the electrification of the Force. Juan believes the Army and Department of Defense (DoD) should explore how to transition to a Force that adopts electric tanks, aviation and transportation vehicles. Moving away from an internal combustion engine has been realised with Tesla, the electrified Chinese and European rails and the electric helicopter. There must then be a source of power for this electrification of transportation and the use of lasers in directed energy weapons and 3D printing at scale. Small modular mobile reactors can provide this power more efficiently than liquid hydrocarbon fuels.
Maintaining the technological edge is also critical to winning wars in the future.
Adopting small modular mobile reactors is already in motion. The Office of the Secretary of Defense Strategic Capabilities Office, which is separate from the military branches, are working on a microreactor prototype. They have already submitted a Request for Information and Request for Solution and are currently in the process of selecting a private company. A few designs will be chosen, which will undergo a 1 to 2 year review process. Building will then begin with the goal of producing a working prototype by 2024, which can be tested and operated in the field to provide power.
Protecting the reactor from livefire in or near a battlefield requires placing it underground where the earth can act as a shield. Further, the Army requires the use of tri-structural isotropic (triso) fuel, which is encapsulated so fission products are unable to escape. In the event the reactor is hit by livefire, no volatiles are able to escape, meaning no cleanup is required. Triso has already been tested thoroughly, making it the preferred fuel choice for quickly building and testing the prototype.
Roadblocks to faster development (38:00)
38:00-42:53 (Juan explains that regulations, siting and environmental assessments slow development.)
Q. What are the main roadblocks which stop faster development?
A. Moving from concept to prototype takes time. Understanding the safety issues is also not easy. The regulations, siting and environmental assessments all take time. However, considering that the Department of Energy (DoE) has estimated that a 50 to 300 megawatt small modular reactor will not be constructed for 10 to 15 years, it is remarkable that the Army will deliver a prototype by 2024.
The Army Reactor Office will help determine where and how the reactor will be placed. Because it will be built at Idaho National Labs, it will be exempt from the usual nuclear regulations. The NRC, however, will still be overseeing the project. The project is currently well-coordinated and moving quickly. For Juan, the project is personal. Adopting nuclear will reduce the number of casualties associated with current fuel transportation, something Juan is passionate about pursuing.

1) Lane's initial interest in nuclear and his college coursework that led him to teaching at Virginia Commonwealth University.
2) He worked, interned, and conducted research all over the field of nuclear in startups and laboratories, but eventually found his place in academia.
3) Lane encourages people to advocate for nuclear and talks about lessons he passes onto his students.
4) Lane discusses the future of nuclear domestically and around the world.
Lane’s Early Career (1:47)
(1:47-14:36) Lane discusses his initial interest in nuclear and his college coursework that led
him to where he is now.
Q. How did you get into nuclear?
(1:47) Lane Carasik is an Assistant Professor of Mechanical and Nuclear Engineering at Virginia
Commonwealth University. When Lane was in high school, he took classes at the local
community college. There one of his math professors revealed that he’d been in the nuclear
field for over 20 years. When Lane began looking into bachelor’s degrees, his mentor
recommended he look into nuclear programs. Lane was interested in nuclear and the benefits
for the environment and decrease the effects of climate change. Lane got into University of
Tennessee-Knoxville where he majored in nuclear engineering.
Q. What about nuclear made you fall in love with the field?
(3:08) Lane says the field of nuclear is so diverse in the different applications and operations.
He also met other people who, after getting nuclear engineering degrees, went onto work in
business or finance. So, the degree seemed specialized, but also broad and useful no matter
where Lane ended up.
Q. What was your PhD in?
(4:40) Lane got his PhD in nuclear engineering focusing on thermal fluids - how to get the heat
from the reactor to the power conversion cycle. His first year of the program was filled with
nuclear engineering classes, but subsequent years he took mechanical engineering, aerospace
engineering, and civil engineering courses.
Q. How did aerospace and civil engineering interact with your PhD in nuclear?
(5:07) To Lane, nuclear engineering is the ultimate interdisciplinary degree. So, to him, all of the
other courses could be extended to apply to nuclear.
Q. During that time you also interned as well?
(5:56) Between community college and his bachelor’s degree, Lane did a research experience
at Georgia Tech Savannah. At the time he got the opportunity to work on Boeing 787s in
Charleston, South Carolina. The next summer he worked at the Tennessee Valley Authority, a
utility partially owned by the government that is otherwise a contractor operator.
Q. After getting your PhD in what was essentially heat transfer, was there anything you felt you
were uniquely an expert?
(12:48) Lane hesitates to use the term expert, but if he had to pick, he would say he specialized
in computational modeling, specifically high-fidelity computational fluid dynamics and design
level fluid dynamics.
Home in Labs and Universities (14:37)
(14:37-16:56) Lane talks about how he experimented with working in different parts of the
nuclear industry but has happily found his place in academia.
Q. After college you moved into the startup world?
(14:37) Lane bounced around to different types of organizations in the industry, but he
eventually came to the realization that national labs and universities was where he wanted to
grow his career. Lane recognizes that both unity and vendors do important work, but it’s now
where he shines.
Q. Why do you shine more in the academic setting?
(16:12) Lane has a lot of ideas - some of them are bad - but a lot of them are good and he loves
getting into the details of research and seeing where it takes him.
Advocating for Nuclear (16:57)
(16:57-49:47) Lane encourages people to advocate for nuclear and talks about lessons he
passes onto his students.
Q. What if national priorities regarding nuclear are wrong?
(16:57) Lane says if we want to realign priorities regarding a national energy policy in the United
States, more people need to advocate to their Congresspeople, their Senators, their House
members, the White House and say ‘we need an energy policy that includes nuclear, that
includes these other energy sources, and this is why.’ What your reasons are, is entirely up to
you. For Lane, he believes we need to prevent climate change from destroying our planet and
having major catastrophic consequences on our society. Lane wants nuclear, but he also
believes in diversifying the energy profile with solar, natural gas, hydro.
Q. How do you think you developed the skills of being both a technical speaking engineering
and also an eloquent policy advocate?
(23:11) Lane’s family has pushed him to expand his abilities beyond the STEM field, especially
in the arts. He is interested in arts associated with construction, for example looking into building
design and architecture.
Q. What lessons have you learned in your early career you share with your students?
(26:00) One of the first lessons Lane teaches his students is to make the connection between
theory and design. Find out if your design is possible using theory. Step two is finding the most
favorable option and proceeding with design. But even then, asking “Will the physics of the
design will work? Are the materials available to be purchased? Is there appropriate pressure
and heat correlations?”
Exploring Fluids and Advanced Systems Technology (49:48)
(49:48-1:03:35) Lane discusses different types of nuclear he researches.
Q. What are some of the challenges you personally want to take on in the transfer space?
(49:48) Lane’s research group is in fluids and advanced systems technology (FAST) and right
now the main focus is the thermohydraulic of an advanced reactor: sodium FAST reactors,
molten salt reactors of either solid fuel or molten fuel, and gas reactors. Lane is interested in all
of them and the tools are similar across the three, primarily only the modeling changes.
Q. Before computers engineers would implement technology with only practical application
knowledge, but now we keep going deeper and deeper into understanding something we’ve
already found the practical applications of, why is that? Do we go too far sometimes, paralyzing
ourselves from making progress?
(54:40) Lane said innate curiosity is one reason. There’s so much left to be learned and so
much more meaningful research to be done.
Q. What’s your favorite coolant fuel combination?
(1:00:18) All of them have fun things going on, according to Lane, but his favorite is molten
salts. They’re inherently cool because they’re a coolant salt of a fuel salt or it can have uranium
or plutonium fuel dissolved into it. For salts in a lab, Lane would probably pick a chloride salt.
Future of Nuclear (1:03:36)
(1:03:36-1:08:19) Lane discusses the future of nuclear domestically and around the world.
Q. What do you think is the future of nuclear?
(1:03:36) Lane believe that if one advanced reactor company is successful in building a new
type of nuclear reactor all companies will benefit. Bret agrees that if one reactor company can
prove a new reactor design works, there will be a rush of investment flow. Nuclear is important,
Lane believes that we need to save the world from climate change. He thinks China and Russia
are going to continue to build reactors and other countries are going to continue buying
reactors, whether that’s from the US or other nations. Lane is also optimistic South Korea will
resume building nuclear reactors and hopefully Japan too. But here in the US, a lot of success
is going to depend on policy changes.
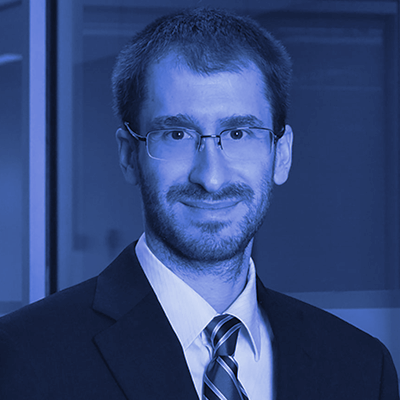
1) How nuclear material neutrons are measured to verify amounts
2) The difference between plutonium-239 and plutonium-240 in the context of nuclear weapons
3) Life in the UAE as a nuclear postdoc
4) Decreasing the attractiveness of plutonium for weapons use with neptunium
Choosing the unique specialty of nuclear engineering (1:02)
1:02-6:50 (Braden explains why he transitioned from a math to a nuclear engineering and why he chose to study at Texas A&M.)
Q. How did you first become interested in nuclear energy?
A. Braden focuses on security and radiation measurements at Virginia Commonwealth University. Braden was originally a math major but switched to engineering because he wanted to solve real world problems. Braden specializes in nuclear engineering because he wanted to focus on something unique.
Braden attended Texas A&M for all three degrees. Braden grew up in Southern California and had considered UC Berkeley, but chose Texas A&M because the university has two test reactors and courses listed under nuclear engineering. He focused his Master’s on nuclear materials but wanted to switch his focus for his PhD to nuclear nonproliferation and radiation measurements. Nonproliferation is making sure that nuclear materials do not become nuclear weapons. Braden thinks of it as stopping the spread of nuclear weapons and nuclear weapons technology.
Measuring neutrons in nuclear materials (6:51)
6:51-9:41 (Braden explains his PhD focus and how neutrons are measured to account for all nuclear material.)
Q. What was the focus of your specific research during your PhD?
A. Braden looked at safeguards, specifically measuring neutrons as a way to account for all nuclear materials. This is used to verify the stated amounts of materials, such as plutonium. This is done by measuring the radiation signature of the neutrons and the gamma rays emitted from the plutonium. Other methods are used in combination, such as radioactive decay to measure the amount of heat emitted. Devices are used to measure gamma rays, but a higher efficiency is needed to measure neutrons, so they are measured isotropically, or in all directions, so the material is encased in a detector. Measuring plutonium is safe, but handlers wear detectors to measure the dose they are receiving.
Reactor versus weapon plutonium (9:42)
9:42-18:43 (Braden explains the difference between plutonium-239 and plutonium-240. He also discusses )
Q. Does plutonium-239 have a different amount of spontaneous fission events than plutonium-240 and are you able to calculate the ratio between the two using the detectors?
A. Yes, there is a different number of spontaneous fission events between the two. Generally, neutrons tell the amount of plutonium-240, so if the entire material was plutonium-240, this would be the mass. Each plutonium isotope emits a different gamma wave energy, so this can be used to calculate a relative concentration. Plutonium’s Z, or atomic number, is 94, meaning plutonium has 94 electrons and is fairly dense.
The majority of the plutonium in the world is trapped in used fuel. Plutonium as an element is also separated from nuclear waste when it is repurposed. This could then be used to create nuclear weapons. Nuclear weapons production required the ratio of plutonium-240 to be minimized. The weapons grade ratio is about 5 percent plutonium-240 and 95 percent plutonium-239. Reactor grade has a higher percentage of plutonium-240. Researchers are reminded not to explore too much into the exact weapons grade because the information does not want to be widely distributed. The government approves 80 percent plutonium-238, but a reactor is not able to go below this. Braden is attempting to quantify the usefulness of specific plutonium isotopes for weapons use through heat transfer calculations to show that some isotopes can deform weapons. His goal is to reduce the attempts to use plutonium in weapons.
Braden’s experience in the UAE (18:44)
18:44-30:44 (Braden explains why he worked in the UAE and his experience there.)
Q. You had an interesting experience in the UAE?
A. After graduating from Texas A&M, Braden worked at the Nevada National Security site but realized he wanted to return to academia. Braden knew somebody at Khalifa University in the UAE in nuclear engineering who told Braden that the department needed a postdoc with experience in radiation measurement. Braden decided to take the opportunity to not only return to academia, but gain experience living in another country.
Braden saw both advantages and disadvantages of living in the UAE. One advantage Braden experienced was the stronger work-life balance present in the UAE. For example, when Braden’s daughter was born, his boss told him to take off work until he felt ready to return rather than giving Braden a set period of time. An interesting thing to note was that many people in Abu Dhabi spend little time outdoors to avoid the heat and dust. Additionally, only 20 percent of the population is from the UAE, while 80 percent are foreigners who make up the workforce, including nuclear engineers and researchers. When Braden asked locals about their views on climate change, they would respond that the UAE leaders were making good decisions, so individuals did not have to make decisions. Braden saw that women used education as a way to gain independence. Women who pursued a higher education could avoid marrying at an early age. Once they have graduated with a PhD, they had more ability to determine when they got married. Braden noticed that female students averaged higher grades than the male students.
Braden returned to the US after the position ended. He was also ready to return because he found that because there is more time spent on enjoying life, productivity in the workplace is lower than in the US. This means that the number of publications an academic will put out is lower, meaning resumes are less competitive when finding jobs in other countries. Braden then followed the path to Virginia Commonwealth University and has been there for three years.
Inadequate high school preparation (30:45)
30:45-37:59 (Braden discusses how his teaching has been challenged by new students who have not been adequately prepared in high school.)
Q. What are some of the things you focus on when teaching?
A. Braden has identified that a challenge is when students transition from high school to college because the rigour in high schools has lessened over time. Students are having an easier time passing high school, meaning they can spend less time studying and still graduate. This produces college students who do not have basic mathematics skills. This leads many engineering students to change majors and not graduate with an engineering degree. Braden has noted that students who take time off after high school to gain real world experience put more time and effort into their engineering degrees because they have a better understanding of why they want to become an engineer. Braden focuses on keeping firm in his decision to not lessen the requirements to pass his engineering courses and believes high schools must improve preparing students for college.
Reducing nuclear material attractiveness (38:00)
38:00-43:55 (Braden explains how he primarily teaches nonproliferation and nuclear security. He discusses how neptunium can be used to pollute fuel and decrease the ability to use plutonium for nuclear weapons.)
Q. What subjects do you focused on in your teaching?
A. Braden likes to teach what he researchers, including nonproliferation and nuclear security. Lately, Braden has been interested in nuclear material attractiveness. He researches how attractive it is to turn a material into a nuclear weapon and if there are quantifiable ways to reduce the attractiveness. The main ways to create plutonium-240 is the amount of fissions that occur in the fuel, known as burnup. The longer the fuel is in the reactor, the more the isotopes shift away from desireable weapons grade plutonium. Braden is devising a way to pollute the fuel in such a way that does not impact operation economics but encourages proliferation resistance. Adding neptunium to the fuel may work because neptunium captures a neutron, creating plutonium-238, which is not good for weapons use. Neptunium comes from recycling fuel. Currently, though, fuel fabrication facilities only work with uranium, meaning there will be infrastructure costs to implement this. Additionally, Braden has noticed that people care more about economics than proliferation.
A future of solar versus nuclear (43:56)
43:56-47:18 (Braden explains how he foresees a future of solar energy improving and becoming nuclear’s primary competitor. He also discusses nuclear’s primary benefits.)
Q. What is your take on the future of nuclear energy?
A. Braden thinks there are a lot of unknowns. Solar is a primary competitor and is improving rapidly. Braden notes, though, that nuclear energy has advantages that the energy market does not consider. The first is the environmental benefit. The second focuses on decreasing the need for energy imports, which increases a country’s vulnerability. Producing all needed energy decreases a country’s reliance on another state. Reliability is the third, meaning power can still be generated during severe weather events.
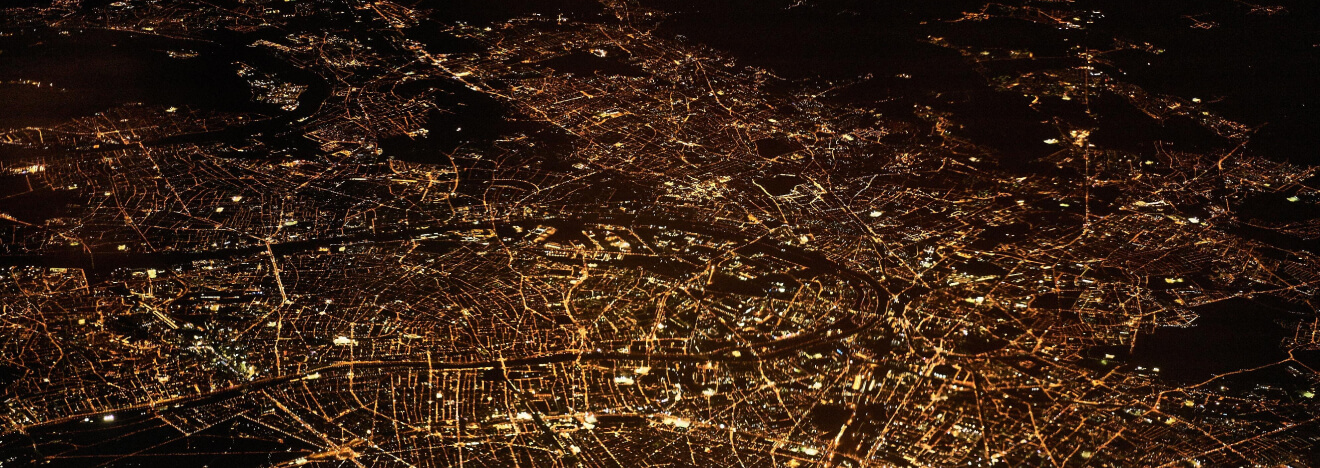
Sign up for our newsletter
No results found
Please try different keywords