TITANS OF NUCLEAR
A podcast featuring interviews with experts across technology, industry, economics, policy and more.
Latest Episode

1) The beginning of Brian’s career and his time in the United States Navy as a diver, as well as what drew him to engineering and nuclear
2) Brian’s initial journey to Oregon State and all of the research projects he’s had a hand in since then
3) Fostering a passion for nuclear in the next generation of nuclear engineers and why the researchers as just as important as the research itself
4) What challenges and successes the nuclear industry will face in the coming years and how to form your individual opinion on nuclear energy
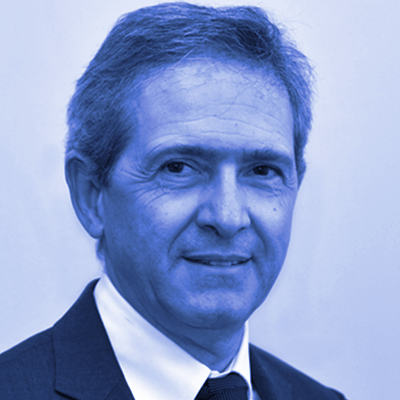
1) Fast reactor coolants and Russian deployment
2) Italy’s strong nuclear education despite the end of the state’s industry
3) The nonelectrical applications for reactor power, including hydrogen production and cogeneration
4) How SMRs take advantage of economies of series, rather than economies of scale, to decrease cost
Stefano enters the nuclear industry (0:07)
0:07-5:24 (Stefano explains how he first got involved with the nuclear industry and his first job after university.)
Q. Where did you get your nuclear experience?
A. Stefano Monti developed his interest for nuclear while a high school student in the 1970s. At the time, Italy was engaged in the nuclear sector and had four power plants in operation. Italy also had a broad research and development (R&D) program, including focus on advanced technologies, and planned to produce 20 gigawatts of electricity from nuclear power. Stefano was attracted to the fact that the nuclear industry was always put in a European or global context, emphasizing international work. Stefano graduated from university in 1982 and then worked for Italy’s largest industrial nuclear company, which focused on developing and designing advanced reactors. Stefano was responsible for the shielding and neutronic design of the material test reactor with a fast neutron spectrum.
Fast reactors (5:25)
5:25-14:25 (Stefano discusses fast reactors, focusing on their coolants and the current fast reactors in operation.)
Q. Let’s talk about fast reactors. What coolant were you working with?
A. In fast reactors, neutrons are moving at faster speeds, and therefore behave differently in the reactor. Water can not be used to bring them to the thermal spectrum, so a different coolant must be used instead. Stefano was working on sodium-cooled fast reactors at the time. Sodium has been used for a while because the physics of sodium cooling is very well known and is less challenging than using other coolants. There are many characteristics to consider when choosing a coolant, the main one being how the neutrons interact with it. Sodium is a good coolant for fast reactors because they only slow neutrons slightly.
There are two industrial sodium cooled fast reactors in operation currently in Russia. Russia has maintained expertise and R&D activity in fast reactor technology. The BN-600, which produces 600 megawatts, has been in operation for 35 years. The BN-800 produces 840 megawatts of electric power. Russia has also developed an alternative fast reactor technology: heavy metal liquid technology. It is a eutectic system, meaning it uses a combination of two different elements to lower the melting point. But there are also downsides; using bismuth produces polonium through irradiation, which emits alpha particles. This requires more safety precautions. However, lead bismuth fast reactors can be used to power submarines.
Italy’s nuclear referendum (14:26)
14:26-20:46 (Stefano explains how Chernobyl occurred during his fast reactor research and how the accident led to the end of Italy’s nuclear program.)
Q. Where did you career progress after working on fast reactors?
A. In 1986, Stefano’s work on the material test for the test reactor was almost complete. However, Chernobyl happened and Italy decided to have a referendum to decide whether to continue with nuclear or not. The Italians where recommended to avoid particular vegetables that had been grown in parts of the country due to contamination from the accident. The politicians recognized that livelihoods of Italian citizens had been impacted and decided to allow the people to have a say in the continuation of nuclear power in the country. The continuation of nuclear also meant the potential for more infrastructure, something the Italian people may not have necessarily wanted when considering the geography of the country.
Italy’s love for complex science (20:47)
20:47-25:37 (Stefano discusses how despite the ended nuclear program, Italy still produces many strong nuclear PhD students.)
Q. Is there a chance Italy may ever go back to nuclear power?
A. Stefano does not foresee a return to nuclear in Italy’s future, despite the number of nuclear PhD students in Italy. This may be because nuclear science is part of Italy’s history. Italy also has a positive and strong attitude towards taking on complex science problems. The possibilities associated with nuclear science foster a creative field that many people are attracted to.
Sharing advanced reactor knowledge (25:38)
25:38-29:52 end of video 1
Q. Do you get particular insight into this because of your job now?
A. Stefano sees his ability to interact with 171 countries as one of the benefits of working for the International Atomic Energy Agency (IAEA). His job as the Head of Nuclear Power Technology Development gives him a broad perspective of what is happening around the world. Stefano is responsible for a program devoted to advanced reactor and nonelectrical applications for nuclear power. One of his main missions is to create a space for the exchange of information. This means the IAEA brings together stakeholders of states participating in advanced reactor technology. One tool they use is the advanced reactor technology information system. This collects detailed information of the Generation 4 reactors, which are the designs for advanced reactors that are not yet in operation, and some Generation 3 reactors that may be in operation. The information system also includes details of the small modular reactors.
Nonelectrical applications: hydrogen production and cogeneration (0:05)
0:05-6:35 beginning of video 2
Q. What are some of the nonelectrical applications of power reactors?
A. Hydrogen production is one nonelectrical application. It can be used to carry energy to create clean transportation. Hydrogen is already produced, but with the byproduct of CO2. Nuclear reactors can be used to extract hydrogen from water using very high temperature reactors or using lower temperature reactors to extract hydrogen from other chemicals.
Cogeneration is another example of a nonelectrical application of power reactors. This is when a reactor is used to generate steam to drive a turbine and the associated heat is used for city heating. China is currently developing a small modular reactor for this purpose. Already 70 nuclear power plants operate cogeneration. However, there are economic considerations that limit cogeneration, such as the need to be competitive with the existing gas or coal plants. Despite the economic considerations, climate change makes nuclear power plant cogeneration a better option because they generate heat without emitting CO2.
Economies of series, not scale (6:36)
6:36-11:13 (Stefano explains why nuclear is not more competitive and discusses how economies of series will decrease SMR cost.)
Q. Why is nuclear not more competitive?
A. The cost structure of nuclear is different to those of fossil fuels. The kilowatt hour is mostly affected by the high investment cost, which makes up between 70 and 80 percent of the total cost. The financial system also manages them differently, meaning there are questions about how to finance nuclear power as building a plant can cost 10 to 20 billion dollars.
Reducing the size of the reactor will decrease the costs associated with building. However, it may not decrease the cost of nuclear produced electricity because larger plants reduce the cost per kilowatt because of economies of scale. However, small modular reactors (SMRs) realize economies of series, which reduce cost and time of construction. This increased manufacturing rate will decrease overall reactor cost.
Stefano’s nuclear future (11:14)
11:14-19:40 (Stefano gives his vision of the future of nuclear.)
Q. What do you see for the future of nuclear energy?
A. Stefano states the expectation for the short or medium term is the have a significant deployment of Generation 3 or higher reactors. The success of new builds depends on the policy and region of the world, however. China and India are expected to deploy these reactors and Russia is expected to see continued development and deployment of advanced reactors. Stefano also foresees SMRs being available within the next 10 to 20 years. SMRs can be used alongside renewables to make up for lost electricity generation during times when renewables can not produce power, such as cloudy or dark days. Stefano also expects newcomer countries to adopt nuclear. States that do not need a high capacity to support their population could adopt SMRs. Because SMRs are yet to be realized, however, Stefano foresees first adoption to take place in more developed countries, such as China. For Europe, Stefano predicts the adoption of nuclear in the hybrid energy system to work alongside renewables and provide nonelectrical applications for industry.
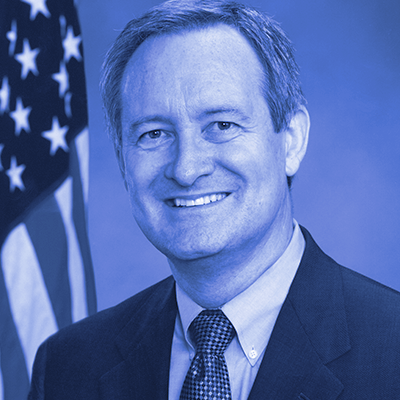
1) Idaho Senator Mike Crapo recounts his childhood in Idaho Falls, Idaho and how the nearby Idaho National Laboratory impacted his career in politics
2) How the economy and the environment can both benefit from win-win energy solutions
3) Crapo provides an overview of the NEICA and NEIMA nuclear energy innovation bills and how they will transform the current nuclear industry
4) Why a whole-of-government approach is necessary for strengthening the U.S. national energy, national security, and economic policies
Growing Up Near Idaho National Lab (0:20)
0:20-6:07 (Idaho Senator Mike Crapo recounts his childhood in Idaho Falls, Idaho and how the nearby Idaho National Laboratory impacted his career in politics)
Q: Tell me about growing up in Idaho Falls, home to the Idaho National Laboratory.
A: Idaho Senator Mike Crapo was born in Idaho Falls, Idaho in May 1951, the same year that the Idaho National Laboratory (INL) first lit a lightbulb with nuclear power at the Experimental Breeder Reactor. Senator Crapo represented the area containing INL in the Idaho State legislature. INL is known for its incredible research and is designated as the nation’s lead nuclear lab today. While Crapo was growing up, the population of Idaho Falls hovered between 40,000 and 50,000 people. At times, the workforce of the lab was pushing a population of 10,000. Everybody in the town either worked at or worked to support the INL.
Senator Crapo was fascinated by politics early on, first getting elected into the Idaho Senate. He enjoyed the policymaking and engagement on issues. Since his district contained the INL, Crapo was always engaged on energy-related issues, including hydropower and nuclear energy. Water is the life blood of any community, especially in Idaho.
Energy, the Environment, and the Economy (6:07)
6:07-10:49 (How the economy and the environment can both benefit from win-win energy solutions)
Q: Over the years, how have you seen your role in terms of negotiating the interface between wilderness, society, and people?
A: Over the last three or four years ago, Senator Mike Crapo has seen a growing conflict between the economy and the environment. There are solutions out there that are better for the environment, clean water, and clean air that are also better for jobs and better for people in the economy. Some of our greatest conservationists are the hunters, fishermen, and farmers. The people who call themselves environmentalists don’t want to drive people out of jobs. The people who have those jobs - the farmers, loggers, ranchers, factory workers - don’t want to ruin the environment. Idaho has been very good at finding solutions that are win-wins.
Senator Crapo joined the Senate in 1998 and is currently serving his fourth term. Crapo previously served six years in the House of Representatives, which is based on majority rules. In the Senate, every Senator has a filibuster right, so every single individual Senator has the ability to push development of policy on legislation. This forces more compromise in which minorities' rights are recognized and dealt with. The Senate has more influence on policy and legislation.
Nuclear Energy Innovation Bills (10:49)
10:49-17:05 (Crapo provides an overview of the NEICA and NEIMA nuclear energy innovation bills and how they will transform the current nuclear industry)
Q: Because of Idaho National Lab’s prominent role in the nuclear world, are you known as a nuclear guy because of your relationship with INL?
A: Senator Mike Crapo is well known for being engaged with nuclear energy. Nuclear power has had trouble with reputation in the past, but that is no longer an issue and nuclear currently has bipartisan support. There was a long period of time - from 1978 to 2012 - when no new nuclear reactors were licensed. It wasn’t because of a lack of interest, but because of reputational and political issues. That process produced a regulatory climate which took years and years to get a license to build a reactor. The cost of that licensing process was so prohibitive that private sector money was increasingly less interested in nuclear. This high cost of nuclear reactors, coupled with the low cost of natural gas, naturally pushed capital to natural gas.
Part of the problem is that a regulator was set up only to regulate, but not to help facilitate the growth of an industry and of a technology. This past year, Senator Sheldon Whitehouse, Senator Crapo, and others came together to pass prominent bills such as NEICA (Nuclear Energy Innovation Capabilities Act) and NEIMA (Nuclear Energy Innovation and Modernization Act). Idaho National Laboratory (INL) does not have a commercial reactor, but fifty research reactors have been built on the site. NEICA aims to create a national reactor innovation center, which has now been built at INL, where the research facilities and research labs are connected with new designs, ideas, and technologies from the private sector. This center directs that the regulator be part of the program so they can learn how to regulate new technologies. The Nuclear Regulatory Commission (NRC) has forty years of experience with light water reactors, but has not been exposed to all the new technologies and developments. This program puts the NRC in a much better position to do new licensing because of their exposure at INL. NEIMA focuses on the Nuclear Regulatory Commission, addressing a number of disconnects in the way that the NRC is mandated and created by law. It directs that NRC provides the private sector with much better guidance and assistance in terms of developing the new nuclear industry.
Nuclear Energy Leadership Act (17:05)
17:05-22:58 (Why a whole-of-government approach is necessary for strengthening the U.S. national energy, national security, and economic policies)
Q: To change a culture of conservatism, you have to go a little bit further so people don’t feel personally responsible if anything went wrong. This prevents people from saying no to everything, even if they know it makes sense intellectually.
A: Senator Mike Crapo is currently working on a bill called NELA (Nuclear Energy Leadership Act). NELA sets a fact that nuclear energy is not just important to energy policy, but also to national security and economic growth. Nuclear energy is a carbon-free force of energy. Every time a reactor shuts down, the replacement fuels for that are carbon-based fuels. Diversified energy and a big part of the portfolio should be carbon-free. Nuclear is the best source of carbon-free energy. Nuclear energy runs the nuclear submarines and aircraft carriers. The ability to have a stable source of energy, without being dependent on other countries, is needed in times of war and jeopardy. This protects U.S. security, but also helps reduce conflict globally and strengthen the U.S. economy.
The NELA legislation directs a whole-of-government approach to accepting nuclear power as a key part of U.S. national energy, national security, and economic policy. Behind the scenes, Senator Crapo and others aim to get a commitment to NELA from those who already committed to NEICA and NEIMA. This will establish a government policy for government-wide priorities that the U.S. will focus on nuclear energy and not let China or Russia take over the leadership of the technology.
Senator MIke Crapo sees the future of the nuclear industry far down the road in the testing and licensing of new reactor technologies that address safety, efficiency, and production issues in much better ways. If these technologies can get licensed, the U.S. could regain ground as a global leader and all the spent nuclear fuel, which has 90% of its power, could be used to generate more electricity. Nuclear could move the U.S. into an incredibly stable source of carbon-free emissions energy that would be a boost to the economy, world standing, and national security.
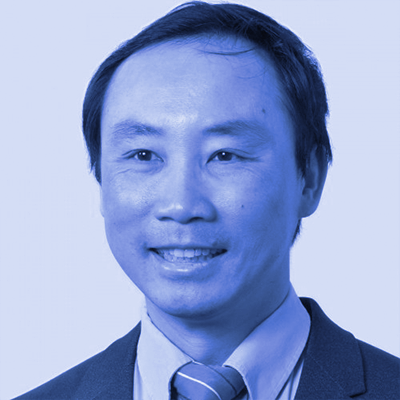
1) Nam Dinh shares his path to nuclear engineering and the international education and research that kicked off his career
2) Why nuclear reactors were not originally designed with failure scenarios in mind and how that has changed
3) Nam reviews how current research projects are changing the way technology and simulation impact the design and licensing of nuclear reactors
Impacts of Reactor Design Modifications (0:19)
0:19-12:50 (Nam Dinh shares his path to nuclear engineering and the international education and research that kicked off his career)
Q: Where did you grow up?
A: Nam Dinh grew up in Vietnam, a country that previously had a nuclear program, but abandoned it due to economic reasons. When the program was still in place, Vietnam held a competition for university entrance. Nam scored very highly and the government sent him on a university scholarship to study nuclear engineering. Nuclear is very exciting for young people, which made it attractive, and people recognize that nuclear requires a lot of mathematical and scientific skills. In 1982, Nam went to study nuclear engineering at the Moscow Power Engineering Institute. He was in the country when Chernobyl happened, a complex time because the country was not very open as a society, leading Nam to focus on nuclear safety. Nam spent six years in Moscow to complete his undergraduate and Master’s degree. He received his PhD and Doctor of Science degrees in Moscow as well.
Nam then went to Sweden’s Royal Institute of Technology for six years; he returned recently and was named Chair Professor of Nuclear Safety. The focus during his first time in Sweden was on severe accidents. Sweden had a different severe accident strategy. When there is a core melt, it will heat up like at Three Mile Island. A majority of Sweden’s reactors are boiling water reactors (BWR). “China syndrome” is a term that describes decay heat eating up the concrete due to a lack of cooling mechanism. Core melt could easily go three meters out or down; the concrete layer is usually one meter thick, so in this case the meltdown could decompose the concrete and get into the groundwater and soil. In the 1960’s and 1970’s, the concept of a severe accident was very modest and the reactors were not designed for such. Sweden decided to put water in the cavity to try to mitigate the core and concrete interaction. However, the addition of water brought up another threat - a steam explosion - which could damage the containment. The Hitachi Economic Simplified Boiling Water Reactor (ESBWR) has a system in which the whole reactor vessel is submerged in the water to cool the vessel and prevent rupture. Without cooling, the system will increase temperature. The gas will put a load on the system and if there is any crack, the fission product can come out. In Europe, Sweden and Switzerland, a system was developed for containment venting. This intentional venting is used with a filter to catch the fission products and prevent a rupture in a severe accident. Understanding aerosols and other containment behavior was much later than the initial design of the reactor.
Nuclear Safety Analysis of Severe Accidents (12:50)
12:50- 26:04 (Why nuclear reactors were not originally designed with failure scenarios in mind and how that has changed)
Q: Why couldn’t the people who originally invented nuclear reactors predict the behavior in the case of a failure?
A: Behavior in a nuclear reactor involves a combination of materials, such as radium dioxide, silicon dioxide, metallic silicon, iron, and fission products. At that time, in the 1940’s and 1950’s, the study of radiochemistry and nuclear material had just started, so the knowledge base was very limited. This knowledge was limited to operation conditions at best, not accident conditions. In nuclear safety, you need to know the limit of your knowledge. The concept of the “worst case scenario” could get very huge, increasing the safety margin and affecting the economics. The engineering aspect is always balancing with uncertainty. Safety is not observable, but must be imagined. However, unsafe conditions, like an accident, are observable. Safety is not the sole issue for nuclear engineering, but the challenge is balancing safety and economics.
According to the current regulator requirements, no one died from Fukushima. However, there were still societal impacts on human health and the land. From that point of view, it was a bad accident. The whole issue is how to manage the very low probability and high consequences risk. The economic consequences of Fukushima were very large and many plants were shut down. Radiation cannot be seen and the risk is perceived very differently. The consequences of shutting down the plants and disrupting production boiled down to the international challenge for nuclear. The question is not as much an engineering issue, but is about much a deeper scientific and societal challenge. If there is a beautiful technology which can make people very happy, but would kill 100,000 people every year, people would not approve of it. However, they do approve of this in the form of the car industry. The question becomes how to make people voluntarily internalize the risk and see the benefit from the risk. In Nam’s research, validation becomes a central question of safety analysis because safety is not observable, so most analysis is done by calculation. This makes the cost of safety very high because engineers have to develop a model through prediction which must validate certain data. There is an intellectual gap between how to model and validate things on such a small scale. In safety analysis, this comes down to uncertainty calculation and safety management.
Role of Advanced Technology in Reactor Design (26:04)
26:04-36:06 (Nam reviews how current research projects are changing the way technology and simulation impact the design and licensing of nuclear reactors)
Q: Should there be an option for companies that bring designs to the NRC to be assessed on a single factor, like proximity to people, instead of assessment based on millions of calculations?
A: A pathway for regulation is available through licensing by testing. Technology is developed, tested, and refined. EBR-II, the Experimental Breeder Reactor, in Idaho was developed for passive safety. This concept is attractive, but is challenging for the vendor. A review process is needed and the NRC convinced of the framework and the process. A single vendor doesn’t have time to do that; they just want the product to be accepted without being involved in the process.
In a reactor design, you must make a safety case by putting evidence together to present. Probabilistic risk assessment is used to do this, but this process takes a long time. Nam Dinh is developing a computerized, formalized safety case as part of his research, similar to artificial intelligence. The computer would represent the safety case in place of a lawyer and can learn a high volume of precedence. If a reasoning can be achieved, the computerized, formalized system can be updated. Engineers may make inconsistent assumptions and a very experienced engineer is usually required to catch these inconsistencies. This computerized, formalized safety case system will make the design and licensing process streamlined and predictable. Many times, unexpected questions may take years to respond. A virtual reactor would allow the system to be tested and able to play with different variations. It then comes down to a question of validation.
In the 1950’s and 1960’s, when the nuclear industry just started, top notch people were teaching nuclear classes at universities. The computer at Los Alamos was developed for supporting nuclear safety analysis and design. New advancing technology, computer science, and modeling simulation bring a new way to look at the same thing. In Nam’s research, he studies boiling heat transfer. This simple concept still has a lot of mystery around it. He measures the temperature and heat flux for an engineering approach and also uses an infrared camera to look into nucleation phenomena. A new advanced simulation can follow each individual bubble and how they interact. Advanced simulation can now go deep into the physics, improving validation, and produces a lot of data very quickly. This is the era of big data and it must be transferred and digested into knowledge by mining the data. Intellectual value and knowledge must be brought into the formation of new technology.
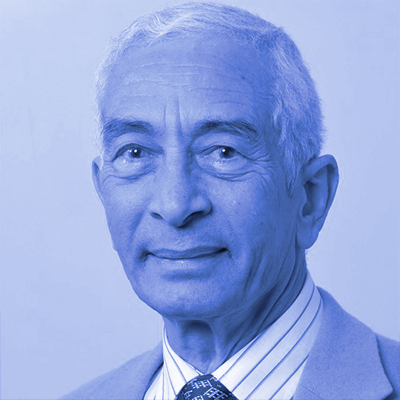
1) Mohamed Bourham’s path to a career in nuclear from Egypt to Europe to North Carolina State University
2) Mohamed reviews his many areas of research, including electrothermal propulsion, radiation, waste, and material science
3) How dry cask storage for spent fuel has performed so far and why there are different ideas about nuclear waste storage
4) Mohamed explains how fusion is controlled with magnetic fields and the impact it has on materials in the fusion reactors
A Nuclear Career Spanning from Egypt to North Carolina (0:48)
0:48-11:11 (Mohamed Bourham’s path to a career in nuclear from Egypt to Europe to North Carolina State University)
Q: When did you first arrive at NC State?
A: Mohamed Bourham first arrived at NC State in Raleigh, North Caroline in 1987. He was born in Egypt, growing up there and starting his career there. Mohamed also spent a lot of time in Europe before coming to the U.S. He received his B.S. in from Alexandria University in Egypt, where all of Egypt’s nuclear professionals get their degree. He then got his Master’s degree from Cairo University and his PhD from Ain Shams University. In June 1965, Mohamed graduated with his B.S. and, by July, was in the Nuclear Research Center of Egypt, a part of the Atomic Energy Authority established in the 1950’s. In October, he was officially appointed as a research engineer in the Department of Plasma Science and Ion Sources. At that time, there was a 2 MW Russian-type research reactor in the Nuclear Research Center. Mohamed was involved in future fusion energy research. A couple years later, the Nuclear Power Plant Authority was created to look into the future production of electricity from nuclear power plants. A site was set aside for the reactor, but the plan was never implemented. The Nuclear Research Center updated their nuclear facilities to a 22 MW research reactor, built under Argentina, and is also used for isotope production for medical uses.
In Europe, Mohamed did plasma and diffusion research in England, Germany, and other countries. When Mohamed came to NC State, they were heavily involved in developing all the techniques needed to support the future of fusion energy. The international system started to show up under ITER and Mohamed became involved with the Department of Energy (DOE). In this program, Mohamed’s students studied problems such as the effect of very high heat flux on components and materials. They became involved with electrothermal plasma sources, which was started with the Department of Defense. This concept allows for propulsion systems and was considered as a pre-injector for rail-guns. He became highly involved in research for the concept and materials needed to survive in this environment, working alongside the Ballistic Missile Defense Organization, Strategic Defense Command, Army Research Laboratory, and the Office of Naval Research.
Mohamed’s Research at NC State (11:11)
11:11-25:21 (Mohamed reviews his many areas of research, including electrothermal propulsion, radiation, waste, and material science)
Q: What is the research, exactly? Are you trying to understand how materials behave or the behavior of the plasma itself?
A: Mohamed and his team understood the impact of the sources and its performance on the integrity of the materials, since it is subject to very high heat, pressure, and exit velocities, up to 7 km/s. This research was taken into the fusion arena because, it they could achieve very high propulsion, it could be used as a fueling mechanism for fusion reactors by accelerating a frozen deuterium to 2-3 km/s so the fuel can get to the core of the fusion reactor before it evaporates. NC State has developed a lot of codes for this, doing all sorts of experimentation and computation. One of Mohamed’s PhD students shuttled between NC State and Oak Ridge National Laboratory, which has an operational electrothermal gun. This student also went to San Diego to go to General Atomics to run experiments on their Tokamak reactor. He was eventually chosen to be a postdoctoral student at ITER, the single individual chosen from the U.S.
Mohamed also took another branch into radiation and waste. NC State got into this research by providing techniques like various codings that provide various barriers and different forms of concrete. Mohamed and his students now have plasma, shielding, and coating as different areas of research. A new contract with the Department of Energy (DOE) looks at non-intrusive sensors that can be used in a nuclear environment, including reactors. The sensors are not inside the reactor, but are outside the reactor and the piezo crystal launches a surface wave which propagates to another exterior detector. Through this pass of the surface wave, measurements of phase change and signatures are detected which are a function of the interior temperature and pressure. The internal conditions being measured are temperature, flow and level of the coolant, and pressure. This project is under a DOE program called NAUP, Nuclear Engineering University Programs. The university can also submit a grant application for equipment, which they have done in the past for a dual ion beam irradiation system and an autoclave stress corrosion.
NC State is also doing a lot of research in materials science. Additional materials, such as lead, could be added to concrete. This has the potential to achieve the same attenuation with a smaller thickness, leading to a reduction in the mass and the cost. Radiation protection is a matter of the specific density of the material. Linear attenuation coefficient is a material-related characteristic, highly dependent on the material. This is transferred into the mass attenuation coefficient, which incorporates the specific density. Mohamed has recently seen a lot of research going on into adding glass components to concrete because of the element of composition. The coefficients are functions of how interactions with the materials take place.
Dry Cask Storage for Nuclear Waste (25:23)
25:23-37:52 (How dry cask storage for spent fuel has performed so far and why there are different ideas about nuclear waste storage)
Q: How do we think about this attenuation coefficient? Is it just a function of the density of the element?
A: The relationship between the coefficients are part of an exponential relationship. The coefficients are functions of how interactions with the materials take place. Most of the focus for waste storage is focusing on the gamma instead of the neutrons. Gamma also produces heat. In the dry cask, there is the spent fuel with an air gap between the fuel and the concrete. The dose rate outside can be calculated to make sure it is within acceptable units. This topic can go very political. Yucca Mountain is a beautiful site as a repository, with a vertical shaft entrance and tunnels with vaults. Most of the spent fuel remains in a large vault on the nuclear power plant site as it cools down before being transported into the casks. The casks are kept within the premises of the nuclear power plant. The repository provides more safety. Most dry casks are performing very well and are a good technique good for 300, 400, or 500 years. Mohamed Bourham does a lot of modeling for dry casks. In collaboration with some colleagues, one of the dry casks was opened after so many years to check for corrosion. Usually, it will not be opened and once it is sealed, it is sealed. During this one inspection, they found that everything looked good and the dry casks performed very well. Other techniques looked at including different concrete or liners to be heat absorbers inside the dry casks.
There is a mix between science, technology, and politics. As researchers, Mohamed and his team look into best thermal barriers, best concrete compositions, and other endless forms of research. NC State talks with Savannah River, Los Alamos, and others like Hanford to provide solutions. It is time now that the world gives a good focus to nuclear waste. It has not been given this much interest in some time. Over the past three years, Mohamed Bourham spent two years working heavily on electrothermal plasmas. One year Mohamed was heavily involved with corrosion studies and different materials used in canisters. He currently has four students studying topics like concrete and concrete forms, coated materials, electrothermal plasmas, and non-intrusive embedded sensors.
Materials and Magnets in Fusion Reactors (37:52)
37:52-47:26 (Mohamed explains how fusion is controlled with magnetic fields and the impact it has on materials in the fusion reactors)
Q: Let’s talk about fusion and materials. Is the Sun a perfect sphere?
A: The Sun’s shape is almost very close to a perfect sphere because it is a gaseous form of hydrogen and has a huge mass, giving it a huge gravitational force. In fusion research, fusion is controlled by a magnetic field instead of gravity. The magnetic field provides a form of a pressure and squeezes the plasma. It also provides a form of confinement and forces the ionized pieces to rotate around the field so they can’t escape. As the magnetic field is applied, the radius gets smaller and smaller and the particles can be controlled. If there was a sudden failure in the magnetic field, called a disruption, all of the particles will find their way inside and through the walls. Mohamed Bourham studies the impact with the materials and the response of the materials to the high heat flux. There are actually three magnetic fields in the Tokamak reactors and there are some features with which one can suppress the disruption so it is not at a high frequency. These magnets are huge and it is an amazing engineering achievement to create these types of magnets. The materials in the Tokamak are important, from the first wall inside to the bottom where the divertor extracts energy and removes ash.
The JET, Joint European Torus, in England is all beryllium inside because it is a very low z material in the beginning of the table and is light. Beryllium’s vapor is very toxic. The test facility built at MIT had tungsten and the one operated by General Atomics has graphite. The study of materials for fusion reactors spread over decades. The interaction from the heat flux point of view and also the impact of the charged particles factor in to the materials. If the reactor is operating using deuterium/tritium mixture, there will be a production of heavy alpha particles and neutrons produced. It is the irradiation that causes material hardening, cracking, and sweating. This is expected to happen in future fusion reactors because there are neutrons. Most of the material research is conducted under the Department of Energy. General Atomics is one of the companies doing a lot of research related to fusion materials and operates their own Tokamak, called the National Fusion Facility. In ten years, the world will start to see the first test reactor that proves the feasibility is there and it can be run and controlled. It can then move into commercialization in the next ten to fifteen years.

1) Jacob Eapen recalls his time working on safety analysis of the advanced heavy water reactor at the Bhabha Atomic Research Center in India
2) How Jacob expanded his career in the U.S. nuclear academic and industrial spaces to focus on computational material science
3) An overview of Jacob’s current and past research projects in the material science space, including analysis of high temperature properties of UO2 fuel
4) Jacob explains why accident tolerant fuels are being developed and some of the challenges faced in choosing a material for the fuel cladding
Safety Analysis of Modified CANDU Reactors (0:46)
0:46-14:17 (Jacob Eapen recalls his time working on safety analysis of the advanced heavy water reactor at the Bhabha Atomic Research Center in India)
Q: How did it all begin for you?
A: Jacob Eapen received his mechanical engineering degree from the University of Kerala in India. While looking for a place to do research, he took the opportunity to work at the Bhabha Atomic Research Center in Mumbai where nuclear reactor research was going on. In India, nuclear is looked at in a very positive manner and is a very prestigious industry, attracting some of the top minds from across the country. Since Jacob had no background in nuclear, he spent the first year in a nuclear training program. He then joined a division called the Division of Reactor Safety, mostly dealing with CANDU-type reactors that went through multiple modifications in India. Jacob worked on an offshoot of the CANDU reactor called the advanced heavy water reactor. In the U.S., the capacity factor has climbed from 91 to 92% and higher over the years. In India, the capacity factor is approaching 90%, but was close to 70% earlier on since there was an emphasis on shutting down reactors.
Jacob starting working on safety codes to model the refueling of a CANDU reactor, which can be refueled without a shutdown. CANDU reactors have long, pressurized tubes. The fuel elements are the shape of short fuel bundles that sit horizontally in the tubes, unlike the vertical fuel assemblies in the U.S. The reactor is heavy water cooled and heavy water moderated. The tubes have refueling machines on both ends which push and receive the fuel. The reactor does not have one pressure boundary like a light water reactor. Since the fuel is smaller, things like flow induced vibrations are minimized and tend to have fewer problems. There is a big neutron field around the reactor, both light water and CANDU. The problems related to fission gas are prevalent in both reactors, but geometry plays a big role. Jacob’s code development focused on accident analysis, including core meltdown.
Computational Material Science in Nuclear (14:17)
14:17-28:05 (How Jacob expanded his career in the U.S. nuclear academic and industrial spaces to focus on computational material science)
Q: When did you come to the U.S.?
A: Jacob Eapen was in the middle of a job search to pursue his Master’s or PhD to do research at the highest level, looking at opportunities in India and the U.S. He chose NC State where he started studying computational flow of plasma dynamics. Mohammed Bourham had a project from the Navy focused on electrothermal plasma launching which Jacob supported from the computational side. Projectiles were thrust to very high speeds, up to 7 km/s. The Navy wanted to get as high as 10 km/s to shoot projectiles to space. Jacob was planning on doing his PhD at NC State, but it was a low point for nuclear at that time in 1998. He took a job to experience the U.S. nuclear industry, leaving NC State to work for Framatome in Lynchburg, Virginia. Jacob worked on many little projects that usually lasted 4-6 weeks, solving client problems related to safety analysis or fluid flow heat transfer. In his two years at Framatome, Jacob learned a lot about the inner workings of the industry, but decided he wanted to get back into academia. Jacob went to MIT for his PhD and his first instinct was to go into thermohydraulics. While there, he learned about material science and had the natural inclination to work on the computational side of materials. The pillars of science used to be just experimental and theoretical. Once the first computers were built, a new pillar evolved which was a mix between theory and experimentation. This computational theory branch takes the concepts from theory and performs experiments on the computer.
One thing Jacob worked on at NC State was graphite. Graphite has a planar structure meaning the carbon atoms are arranged in a honeycomb lattice and are very strongly bonded on a plane. There are many layers of these planes that are loosely tied together. Graphite was the first moderator used by FERMI and Chicago Pipe and was also used by the British. The graphite moderator program in the U.S. in the 1950’s in the aircraft reactor program, which didn’t pan out. In the 1960’s, Oak Ridge took initiative and build a molten salt reactor with a graphite moderator, which ran for a few years. The DOE is currently looking at the possibility of the next generation reactors that can go to higher temperatures to achieve thermodynamic efficiency. Graphite is a great moderator that can withstand high temperatures. The microstructure changes with neutron irradiation and fission gases create big voids in graphite. One of Jacob’s projects was focused on creep of graphite, looking at how different defects were formed in the graphite. When exposed to radiation, graphite expands along one axis and shrinks along another axis.
High Temperature Properties of Uranium Oxide Fuel (28:05)
28:05-44:53 (An overview of Jacob’s current and past research projects in the material science space, including analysis of high temperature properties of UO2 fuel)
Q: What other type of work does your lab focus on?
A: When Jacob Eapen joined NC State in 2008, he was looking for material science projects because he did computational material science in his PhD. Following that, he went to Los Alamos for a short time where he worked with Art Voter, a well-known scientist in the area of accelerated molecular dynamics. After this, Jacob went to NC State. One of his materials projects was on graphite and another project was on high temperature properties of uranium oxide (UO2) fuel, greater than 2,000 degrees Kelvin UO2 has a peculiar property called superionic property. Beyond a certain temperature, one of the ions starts diffusing like a liquid. It is solid state and the uranium is keeping the lattice in place, but the oxygen ions are diffusing in and around uranium pillars. When Jacob and his team started looking at it, they were able to simulate this behavior in the UO2. Simulations are a big help when there are a lot of conflicting theories. He also used a spectroscopic technique to get data about dynamic structure function. Models are used to make sense of this very abstract data.
In the early 80’s, the UK, who were the leaders in neutron scanning techniques, predicted that the oxygens were leaving the lattice. However, they couldn’t fit the placement of the ions into any known models. One person said that the oxygen ions were just jumping from one place to another, not flowing. Jacob Eapen picked this work up and saw that the ions were jumping. This led him to the world of supercooled liquids. When atoms are cooled very quickly, they are in a frustrated state and tend to follow things along a line, in a string-like motion. If the temperatures are raised even higher, it goes to a pseudo phase transition called lambda transition. Around 2,700 degrees Kelvin the specific heat property value shoots up and comes back down, representing a second-order phase transition. Jacob was able to show that, as early as 2,000 degrees Kelvin, the ions slowly start moving from the lattice. At low temperatures, there are a small number of very long strings. As temperature increases, there are more strings but of a shorter nature. Many of the fission products take the place of the uranium, not the oxygen. The thermal conductivity of graphite was also looked at and another student looked at transfers in non-metallic insulators. Jacob does a little bit related to the quantum effects, such as bonding, but Jacob is more interested in the collective nature of atoms.
Cladding Materials in Accident Tolerant Fuel Design (44:53)
45:53-54:44 (An explanation of why accident tolerant fuels are being developed and some of the challenges faced in choosing a material for the fuel cladding)
Q: Are there no quantum effects that are not being accounted for?
A: Jacob Eapen does a little bit related to the quantum effects, such as bonding, but Jacob is more interested in the collective nature of atoms. Material scientists are more interested in the prediction of structures and new structures. There are very good approximations, such as density functional theory. One of his ongoing projects does this, but it is very expensive to complete the calculations. Jacob tries to find the interatomic potential that would mimic the electronic behavior to calculate the forces between two atoms. Quantum effects are smaller at higher temperatures.
Jacob has a project ending soon on accident tolerant fuels. Accident tolerant fuels (ATF) started from Fukushima in 2011 and the Department of Energy (DOE) reorganized the fuel program to include ATF programs. One main focus was how to control hydrogen, which was the big problem with zirconium. An exothermic chemical reaction is happening which produces a lot of heat, which is the downside of the zirconium that makes up the cladding material. Zircaloy does not absorb neutrons like steel, which is why steel is not used in cladding material in light water reactors. Another option for the cladding include silicon carbide composites, which has small cross-sections and does not react with water. Long filaments of silicon carbide are put in a matrix of silicon and carbide, which creates a silicon carbide composite. There is a good chance that this was developed in the 60’s at Los Alamos or Oak Ridge. In the 60’s, there was a tremendous interest by the Navy to develop nuclear reactors for their submarines and aircraft carriers, which pumped a lot of money into nuclear development. At the time, there was a need to replace the noisy diesel generators that could easily be detected by the Russians. That kind of need is not there any more.
Climate change is real and fossil fuels are adding a lot of carbon into the atmosphere and the oceans. A carbon neutral source like nuclear is needed to counter climate change. Other renewable sources cannot be scaled to the level that nuclear can go with current technology. Jacob’s wish is that the next generation of people can bring the next generation of reactors that can scale up.
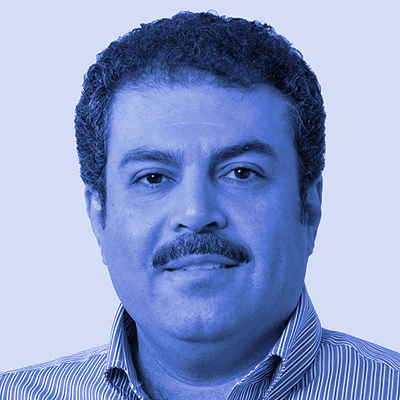
1) Increasing measurement accuracy with gamma rays
2) Understanding cross sections, thermal neutrons and fast neutrons
3) The PULSTAR research reactor design and how it is used to produce positrons
4) Demonstrating inherent safety with small research reactors
Pushing accuracy resolution to a 10th of a percent (0:28)
0:28-8:14 (Ayman explains how he first entered nuclear engineering. He goes on to explain the focus of his PhD research.)
Q. How did you become interested in nuclear engineering?
A. Ayman Hawari first became interested in science as a child growing up in Jordan and soon discovered the field of nuclear engineering. Ayman attended the University of Missouri-Rolla for his undergraduate degree after attending a year of high school in Missouri as an exchange student. He then went on to complete his graduate degrees at the University of Michigan. He is now the Director of the PULSTAR reactor at North Carolina State University.
Ayman focused his PhD on radiation detection and high accuracy measurements. The goal of high accuracy measurements is to increase accuracy resolution to a 10th of a percent. During his PhD research, Ayman and colleagues would hit the limit of how accurately they could measure the quantity of reaction rates. Ayman discovered that the nature of isotopes could be used to improve this resolution, particularly when doing relative measurements of reaction rates, known as spectrum indexes, for the energy distribution of the neutrons in the vicinity of a radiation source. Standard sources were used in the previous technique, which had limited accuracy. Ayman noticed that if the calibration was done differently using specific nuclides, such as cobalt-60, scandium-46 or sodium-24, the cascading gamma rays had intensities relative to each other better than a 10th of a percent. Detectors differentiate the gamma rays coming off of different nuclides in the same area. Gamma spectrometers convert the energy of an incoming gamma ray into electric pulses, which can then be measured for voltage, currents or amplitude. 3D maps can be built based on any type of radiation source, including gamma rays and neutrons, to better see what is happening. Researchers are just beginning to explore whether sensors can be placed around the outside of a reactor to generate maps without placing sensors inside the reactor.
Studying fuel burnup in industry (8:15)
8:15-17:49 (Ayman explains the work he did after completing his PhD, including research on fuel burnup in advanced reactors.)
Q. Where did you take your PhD work to?
A. Ayman looked at utilizing these relative measurement techniques in multiple areas. For example, Ayman looked at the assay of nuclear fuel to better understand burnup and applied this to advanced reactors. This can be done either when the fuel is taken out of a reactor or during its use in a circulating fuel system. In the latter, the sensors could be placed online, allowing researchers to see the characteristics of the flowing fuel. This is important to make sure that consumed fuel is not put back into the reactor because used fuel is highly irradiated and could cause the other fuels to fail. This is important in circulating pebble reactors to control where the pebble fuel flows within the reactor. This type of reactor has been explored overseas. They were built in Germany and one is in operation in China. The US is currently researching how the pebble reactors can meet specific limits set by the regulator. Designers are trying to push these reactors to market because they are more efficient and the electricity production per unit of thermal power is higher than the light water reactor.
Ayman worked in industry before returning to academia. He worked for Combustion Engineering, a vendor, focusing on their light water and boiling water reactors (BWRs). Combustion Engineering also built a fleet of pressurized water reactors (PWRs) in South Korea and worked on BWRs in Sweden. The limitations for both PWRs and BWRs in terms of fuel burnup and detection are similar. Because of the different fuel rod assemblies between the designs, measurements are taken at different places. Detectors can be used to span the radius of the fuel to create a 3D rendering of the fuel burnup distribution. Theoretically, the fuel can be resorted and optimized on a pellet by pellet basis, both only if the pellet radiation damage could be characterized with a high level of confidence. Reprocessing fuel is in existence, but simplifying the process is complex.
Thermal neutrons and cross sections (17:50)
17:50-28:16 (Ayman explains why he returned to academia and discusses his focus on nuclear data, including an explanation of thermal neutrons and cross sections.)
Q. What brought you back to academia?
A. Ayman returned to academia to teach the next generation and to pursue the freedom of research. One area Ayman focuses on now is nuclear data. Calculations require accurate cross sections of radiation interactions. A cross section is related to the probability that a neutron will hit any given atom. Ayman focuses on thermal neutrons, which are relatively low energy and drive most reactor concepts. Computer models are used to model thermal neutrons and study atomic trajectories, or the position of a given atom at a particular moment in time. Atomic trajectories are then used to calculate energy and momentum distributions for a material, which is then used to predict how a neutron may either gain or lose energy. A neutron can pick up energy from a system if it is at low energy, known as upscattering. The research Ayman performed to find the cross section energies of the neutrons in graphite has been shared with the National Nuclear Data Center and is used all over the world.
Researching fast neutrons, which are significantly faster than thermal neutrons, is complicated. This is because the interactions are directly within the nucleus, and researchers know less about the available energy within it. Theoretical calculations are accurate for thermal neutron reactors and estimates are tested and validated through experimentation, but evaluations involving nuclear forces are usually a combination of theoretical data and experimental and measured data.
Understanding cross sections can be done using a mathematical formula. The thermal range uses three terms: energy and momentum distribution (a function), incoming and outcoming energies of the neutrons (a ratio), and the actual nuclear cross section. These terms are multiplied together to reveal the cross section of a thermal neutron. The nuclear cross section of any given element is modeled based on quantum mechanics. Nuclear forces inside the nucleus are still not perfectly known, however. Researchers are working on models to better understand how nucleons interact.
Producing positrons (28:17)
28:17-36:14 (Ayman explains how positrons are generated by the PULSTAR research reactor.)
Q. Tell me more about the types of research projects in your lab.
A. The PULSTAR reactor is a one megawatt research reactor at North Carolina State University. All reactors produces heat and radiation. Heat is the focus of power generating reactors, but research reactors produce significant intensities of neutrons and gamma rays. PULSTAR produces fluxes of fast and thermal neutrons. Both neutrons and gamma rays are harnessed and used in radiation testing, forensic examination of materials to determine isotope makeup, and to build instruments to reveal the atomic components of materials. These instruments include neutron diffraction and positron beams. A positron beam is built after generating positrons when gamma rays are converted into antimatter through pair production. Nuclear reactors produce gamma rays. When they hit a target and interact with electrons, they produce positrons, which are the opposite of antimatter. The target is platinum and a beam tube captures the positrons, which are then extracted from the tube and injected into matter. A platinum target is used because it is heavy and lasts longer in the intensity of the radiation environment.
PULSTAR (36:15)
36:15-41:37 (Ayman explains the PULSTAR research reactor and how it is different from the TRIGA reactor.)
Q. What else happens in PULSTAR and how is it different from a TRIGA?
A. PULSTAR uses a different fuel, similar to that of power reactors. The enrichment levels are 4 percent and 6 percent. The PULSTAR can help the sector better understand fuel limits by providing operational data. This data can hopefully be used to increase the enrichment levels used in power reactors. The PULSTAR is also designed differently than TRIGA. PULSTAR is designed so that the highest neutron concentrations exist on the periphery of the core. This happens when it is under moderated by adjusting the water and fuel ratio, allowing neutrons to escape from the core. This allows neutrons to efficiently be lifted for experimental use without changing the core for each experiment.
PULSTAR is named as such because a pulse used to be generated by the reactor. A rod would be removed quickly, creating a semi instantaneous reaction rate increase of core fission, creating a pulse. The increase would change from 1 megawatt to 1 gigawatt over about 10 milliseconds. The mechanism that returns the reaction rate to normal is the doppler feedback.
Decreasing regulation for small reactors (41:38)
41:38-53:12 (Ayman discusses how a potential for an autonomous reactor may be slowed due to regulation requirements. He also explains how his work is proving to regulators that smaller reactors are inherently safer than large reactors.)
Q. With all of these feedback loops, do we even need to control a nuclear reactor?
A. Researchers are working on designing autonomous reactors. They are theoretically possible and most likely to be developed first in small reactors. This is because the small reactors have different safety scenarios and consequences from the large reactors. When considering safety, the delayed release of energy, or decay heat, must also be considered. Decay heat is the heat left over after a reactor is shut down and is usually a few percent of the power a reactor generates. In large reactors, this percentage could be high enough to melt structural systems, requiring additional safety measures to be put in place. This risk does not exist in smaller reactors because the decay heat is low. Demonstrating this low risk could lead to reduced regulation. While this is supported, the change in regulation is taking a while to occur. This might be because of the potential to impact a populated area, triggering the industry to be overly careful. Ayman’s work shows that research reactors under a certain power level are inherently safe, meaning regulation may become less strict in the future. Ayman sees nuclear energy as a necessity for human progress. He finds it exciting because there are still things to be discovered, creating a great area of study for students. Ayman believes the future of nuclear is bright.

1) The difference between plasma and gas
2) How fusion differs from fission
3) How plasma enables low heat molecule deconstruction
4) The current and future uses of plasma for industry manufacturing and advancements
How Steve ended up studying plasma physics (1:13)
1:13-13:52 (Steve explains how his interest in science stems from his father. He also gives an introduction to the difference between fission and fusion events.)
Q. Where did your interest in nuclear begin?
A. Steve Shannon contributes his interest in science to his dad who was a self-employed industrial engineer. Steve grew up in Detroit and attended Michigan for both undergraduate and graduate school, earning a PhD in nuclear engineering. He now focuses on plasma physics, which is related to nuclear engineering in the context of fusion energy. Fusion energy comes from the joining of atoms rather than splitting. The mass deficit of combining atoms is released as kinetic energy in the form of helium and a neutron, for example. As they fly off from the fusion event, they create heat. Fusion leverages that heat for two reasons. The first is that the heat can be converted into electrical energy. The second is that the heat can be used to sustain the reaction, which unlike fission, is required in fusion events. The energy produced by the fusion event will sustain the reaction without the need for auxiliary heating, meaning the charged particles will stay in the fusion reaction, creating carryon fusion events. Deuterium and tritium are the isotopes of hydrogen that create the most energy advantageous fusion reactions.
Using plasma in manufacturing (13:53)
13:53-22:39 (Steve discusses what a plasma is and how it can be used to create such things as computer chips and solar panels.)
Q. Where did you take your studies when you decided to research plasma?
A. The difference between a gas and a plasma is a plasma collectively responds. Plasma is ionized gas, meaning the electrons have been torn off the atom to produce positively charged ions and electrons. Ionization is the process of separating the charge from the nucleus.
After graduate school, Steve worked on using plasmas to manufacture computer chips. This process involves the use of electrons and ions in a plasma to modify silicon and other materials. Using plasma creates the ability to control the direction in which a chemical reaction occurs. Because plasmas have a large mass difference between the electrons and ions, heat can be controlled, meaning only the electrons within the plasma are heated. This is beneficial in the creation of film solar cells. Silane gas (SiH4) is heated to deconstruct the molecule, removing the silicon from the hydrogen. The silicon attaches to the glass, creating solar panels. Heating only the electrons enables the molecular deconstruction without heating the entire gas to dangerous temperatures.
Plasma advancements accelerate industry developments (22:40)
22:40-32:22 (Steve explains how plasmas are created. He also discusses more industrial plasma applications.)
Q. Do plasmas only work with gases?
A. Plasmas are the natural progressions of heating up an element. With a volume of gas, an electromagnetic field is applied to add electrons, often times using radio waves. Because the gas is at room temperature, Steve can explore depositing silicon on materials like plastic. This means flexible solar panels and electronics are becoming available. There are many practical industrial applications for plasma. Fusion can be compared to the moon landing in that the actual moon landing did not have a great economic output, but the technological innovations that came out of the moon landing were great. Much of the fusion technology has been used for other applications. For instance, innovations in fusion have accelerated the development of electronics, providing us with products including cell phones and laptops.
Returning to academia (32:23)
32:23-37:30 (Steve explains why he returned to academia after working in Silicon Valley. He also discusses what he hopes his students take away from his classes.)
Q. How did return to academia from industry?
A. After graduate school, Steve moved out to Silicon Valley to work at Applied Materials, which is a company that produces the plasma reactor equipment for computer chip production. While undergoing training, Steve started teaching night classes in the chemicals and engineering department at San Jose State University. While Applied Materials supported his teaching, Steve found that he enjoyed working in academia more than industry and he began working at North Carolina State University as a professor of nuclear engineering.
Steve wants his students to be able to work independently. His biggest goal is for his students will show him what they did and explain to him why they did it rather than wait to be told what to do. Steve believes the ability to find one’s own way is what makes a strong researcher.
The future of plasma applications (37:31)
37:31-41:10 (Steve discusses the future applications for plasmas.)
Q. Where do you expect your students will take plasma research in the next 10 to 15 years?
A. There are many things about the plasma environment that are still not well understood. Much is still to learn before plasmas can be modeled more accurately. Modeling will become more important as new industrial applications for plasma are discovered. Plasmas are already starting to be used in agriculture and medicine, such as for cancer treatment. For example, because plasma produces the same chemical makeup generated by cells under stress, it can be used to accelerate the body’s stress response, such as increased blood clotting. Once the level of understanding associated with computer chip production is reached in other areas, a wide new array of applications can be developed.
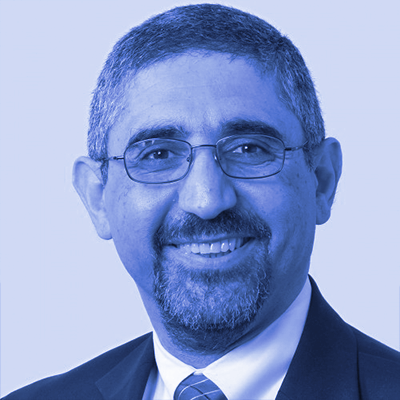
1) Yousry Azmy recalls his path to academia in the nuclear space and explains how neutrons interact in a nuclear reactor
2) Yousry Azmy discusses his research in solving the transport equation on unstructured grids and explains the levels of a reactor transport problem
Neutron Interaction in a Nuclear Reactor (0:30)
Video P1 0:30-14:59 (Yousry Azmy recalls his path to academia in the nuclear space and explains how neutrons interact in a nuclear reactor)
Q: Where did it all begin for you? How did you know about nuclear?
A: Back in Egypt in the late 1960’s and early 1970’s, Yousry Azmy was finishing high school and decided that engineering was where his heart was. In Egypt, the education system is a little bit different. In a five year system, the first year is preparatory where everyone takes the same classes. During this year, Yousry stayed in Cairo to study mechanical engineering, but later decided he didn’t want to do mechanical and moved mid-semester to transfer to the nuclear engineering program in Alexandria. Yousry was drawn to nuclear because of the rigor and good mix between technology and basic science. The mathematically and computationally-oriented studies appealed to Yousry from the early stages. Nuclear was a bit abstract, which also appealed to his type of thinking and attitude.
Yousry Azmy came to the U.S. in 1980 to pursue his graduate program at the University of Illinois-Urbana Champaign, receiving his Master’s in 1982 and his PhD in 1985. The focus of his career has been transport theory. Yousry’s three-piece dissertation was on basic math of numerical methods, CFD (computational fluid dynamics), and transport, primarily diffusion acceleration, which is a way of solving the transport equation faster under certain conditions. Radiation has three features that characterize it: space, the energy of the particles, and the direction of their flight. There are two major classes of methods for angles: spherical harmonics and discrete ordinates, which is Yousry’s focus. Sit at any point in the reactor and there will be neutrons flying in every possible direction. Beam tubes create a path of least resistance that neutrons are not going to interact with much in a containment that is either non-absorbing or non-permitting. It does not prevent the waste from the neutrons that don’t make it in. They are just reducing the number that are not going to make it by reflecting them into the path.
Neutrons are born in fuel pins at very high energy because fission produces very high energy neutrons, a majority in the meV range. In light water reactor technology, water is used because hydrogen happens to slow them down quite a bit. The probability of the neutrons having an interaction with fuel to generate even more neutrons and sustain the chain reactor increases with decreasing energy in the thermal range. Neutrons are born at fission energy and, if they aren’t slowed down, there is a very little likelihood they will produce more fission and sustain the chain reaction. The slower the neutrons get, the higher the probability that they would interact. There are certain minute differences in the way a neutron interacts with the nucleus. Fission occurs in the first place because, once the neutron is absorbed, the compound nucleus is excited and needs to stabilize by ejecting energy.
Transport Theory Calculations on Unstructured Grids (0:15)
Video P2 0:15-9:47 (Yousry Azmy discusses his research in solving the transport equation on unstructured grids and explains the levels of a reactor transport problem)
Q: Does your lab focus on things other than transport?
A: Yousry Azmy’s lab does transport and applications of transport if it happens to be a heavy lift problem numerically or computationally. One of the big projects he has in his group is a new code for solving the transport equation on unstructured grids. People in computational fluid dynamics (CFD) have done this for ages, for example, in the design of aircraft wings. In neutronics, there are a lot of curved surfaces, such as a fuel pin and control rod. The method of long characteristics does not require discretizing into a spatial mesh. The pins and cladding are kept as curved surfaces and the problem is solved using the method of long characteristics and homogenization to represent a fuel assembly as a block without the inside of it. Unstructured grids are not needed in reactors at this stage, but in 5-10 years from now when quantum computing becomes a reality, people may want to do that. In applications like medicine, unstructured grids may be needed to capture the detail of a bone, tissue, or the surface of a tumor.
There are three levels of doing a reactor transport problem. The level of the actual reactor is done with diffusion theory, which is a simplified version of transport theory. The previous level is the assembly level, which is done with transport, on a single assembly at a time. The input to the intermediate level comes from another transport solver prior to that, which deals frequently with individual fuel pins or clusters of pins to reduce the number of energy groups. Yousry’s codes are mostly for research, but there is a code system at Oak Ridge National Lab that is available for engineers. Universities are not designed to do this type of production. They look at the next big thing, not the current big thing.
Currently, the major thrust in research is in nuclear security and non-proliferation. The transport solver is an engine that could be attached to anything you want to drive. If the obstacle in the next design is one portion of the transport calculation, Yousry will then go out to surmount this obstacle by thinking of something novel and put his students on it for their PhD’s. The nuclear space is very difficult to predict because of the speed at which computational speed is evolving. In the next few years, the GPUu technology is going to see continuous growth. The push towards exascale computing is going to make a big splash when it happens. The question for applications people is where they can carve a niche and exist in it as nuclear computational scientists. The unpredictable wild card is quantum computing which will change everything.
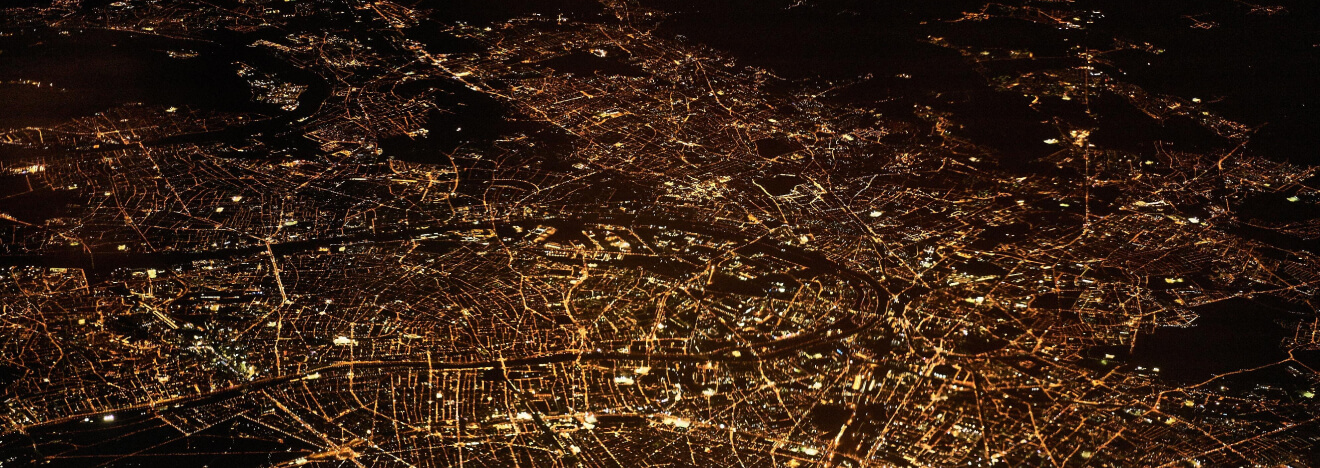
Sign up for our newsletter
No results found
Please try different keywords