Ep 232: Ayman Hawari - Director, PULSTAR reactor, North Carolina State University
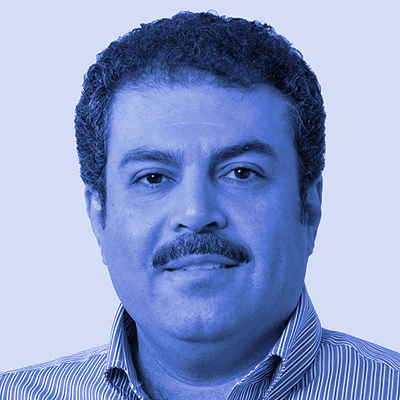
Show notes
Pushing accuracy resolution to a 10th of a percent (0:28)
0:28-8:14 (Ayman explains how he first entered nuclear engineering. He goes on to explain the focus of his PhD research.)
Q. How did you become interested in nuclear engineering?
A. Ayman Hawari first became interested in science as a child growing up in Jordan and soon discovered the field of nuclear engineering. Ayman attended the University of Missouri-Rolla for his undergraduate degree after attending a year of high school in Missouri as an exchange student. He then went on to complete his graduate degrees at the University of Michigan. He is now the Director of the PULSTAR reactor at North Carolina State University.
Ayman focused his PhD on radiation detection and high accuracy measurements. The goal of high accuracy measurements is to increase accuracy resolution to a 10th of a percent. During his PhD research, Ayman and colleagues would hit the limit of how accurately they could measure the quantity of reaction rates. Ayman discovered that the nature of isotopes could be used to improve this resolution, particularly when doing relative measurements of reaction rates, known as spectrum indexes, for the energy distribution of the neutrons in the vicinity of a radiation source. Standard sources were used in the previous technique, which had limited accuracy. Ayman noticed that if the calibration was done differently using specific nuclides, such as cobalt-60, scandium-46 or sodium-24, the cascading gamma rays had intensities relative to each other better than a 10th of a percent. Detectors differentiate the gamma rays coming off of different nuclides in the same area. Gamma spectrometers convert the energy of an incoming gamma ray into electric pulses, which can then be measured for voltage, currents or amplitude. 3D maps can be built based on any type of radiation source, including gamma rays and neutrons, to better see what is happening. Researchers are just beginning to explore whether sensors can be placed around the outside of a reactor to generate maps without placing sensors inside the reactor.
Studying fuel burnup in industry (8:15)
8:15-17:49 (Ayman explains the work he did after completing his PhD, including research on fuel burnup in advanced reactors.)
Q. Where did you take your PhD work to?
A. Ayman looked at utilizing these relative measurement techniques in multiple areas. For example, Ayman looked at the assay of nuclear fuel to better understand burnup and applied this to advanced reactors. This can be done either when the fuel is taken out of a reactor or during its use in a circulating fuel system. In the latter, the sensors could be placed online, allowing researchers to see the characteristics of the flowing fuel. This is important to make sure that consumed fuel is not put back into the reactor because used fuel is highly irradiated and could cause the other fuels to fail. This is important in circulating pebble reactors to control where the pebble fuel flows within the reactor. This type of reactor has been explored overseas. They were built in Germany and one is in operation in China. The US is currently researching how the pebble reactors can meet specific limits set by the regulator. Designers are trying to push these reactors to market because they are more efficient and the electricity production per unit of thermal power is higher than the light water reactor.
Ayman worked in industry before returning to academia. He worked for Combustion Engineering, a vendor, focusing on their light water and boiling water reactors (BWRs). Combustion Engineering also built a fleet of pressurized water reactors (PWRs) in South Korea and worked on BWRs in Sweden. The limitations for both PWRs and BWRs in terms of fuel burnup and detection are similar. Because of the different fuel rod assemblies between the designs, measurements are taken at different places. Detectors can be used to span the radius of the fuel to create a 3D rendering of the fuel burnup distribution. Theoretically, the fuel can be resorted and optimized on a pellet by pellet basis, both only if the pellet radiation damage could be characterized with a high level of confidence. Reprocessing fuel is in existence, but simplifying the process is complex.
Thermal neutrons and cross sections (17:50)
17:50-28:16 (Ayman explains why he returned to academia and discusses his focus on nuclear data, including an explanation of thermal neutrons and cross sections.)
Q. What brought you back to academia?
A. Ayman returned to academia to teach the next generation and to pursue the freedom of research. One area Ayman focuses on now is nuclear data. Calculations require accurate cross sections of radiation interactions. A cross section is related to the probability that a neutron will hit any given atom. Ayman focuses on thermal neutrons, which are relatively low energy and drive most reactor concepts. Computer models are used to model thermal neutrons and study atomic trajectories, or the position of a given atom at a particular moment in time. Atomic trajectories are then used to calculate energy and momentum distributions for a material, which is then used to predict how a neutron may either gain or lose energy. A neutron can pick up energy from a system if it is at low energy, known as upscattering. The research Ayman performed to find the cross section energies of the neutrons in graphite has been shared with the National Nuclear Data Center and is used all over the world.
Researching fast neutrons, which are significantly faster than thermal neutrons, is complicated. This is because the interactions are directly within the nucleus, and researchers know less about the available energy within it. Theoretical calculations are accurate for thermal neutron reactors and estimates are tested and validated through experimentation, but evaluations involving nuclear forces are usually a combination of theoretical data and experimental and measured data.
Understanding cross sections can be done using a mathematical formula. The thermal range uses three terms: energy and momentum distribution (a function), incoming and outcoming energies of the neutrons (a ratio), and the actual nuclear cross section. These terms are multiplied together to reveal the cross section of a thermal neutron. The nuclear cross section of any given element is modeled based on quantum mechanics. Nuclear forces inside the nucleus are still not perfectly known, however. Researchers are working on models to better understand how nucleons interact.
Producing positrons (28:17)
28:17-36:14 (Ayman explains how positrons are generated by the PULSTAR research reactor.)
Q. Tell me more about the types of research projects in your lab.
A. The PULSTAR reactor is a one megawatt research reactor at North Carolina State University. All reactors produces heat and radiation. Heat is the focus of power generating reactors, but research reactors produce significant intensities of neutrons and gamma rays. PULSTAR produces fluxes of fast and thermal neutrons. Both neutrons and gamma rays are harnessed and used in radiation testing, forensic examination of materials to determine isotope makeup, and to build instruments to reveal the atomic components of materials. These instruments include neutron diffraction and positron beams. A positron beam is built after generating positrons when gamma rays are converted into antimatter through pair production. Nuclear reactors produce gamma rays. When they hit a target and interact with electrons, they produce positrons, which are the opposite of antimatter. The target is platinum and a beam tube captures the positrons, which are then extracted from the tube and injected into matter. A platinum target is used because it is heavy and lasts longer in the intensity of the radiation environment.
PULSTAR (36:15)
36:15-41:37 (Ayman explains the PULSTAR research reactor and how it is different from the TRIGA reactor.)
Q. What else happens in PULSTAR and how is it different from a TRIGA?
A. PULSTAR uses a different fuel, similar to that of power reactors. The enrichment levels are 4 percent and 6 percent. The PULSTAR can help the sector better understand fuel limits by providing operational data. This data can hopefully be used to increase the enrichment levels used in power reactors. The PULSTAR is also designed differently than TRIGA. PULSTAR is designed so that the highest neutron concentrations exist on the periphery of the core. This happens when it is under moderated by adjusting the water and fuel ratio, allowing neutrons to escape from the core. This allows neutrons to efficiently be lifted for experimental use without changing the core for each experiment.
PULSTAR is named as such because a pulse used to be generated by the reactor. A rod would be removed quickly, creating a semi instantaneous reaction rate increase of core fission, creating a pulse. The increase would change from 1 megawatt to 1 gigawatt over about 10 milliseconds. The mechanism that returns the reaction rate to normal is the doppler feedback.
Decreasing regulation for small reactors (41:38)
41:38-53:12 (Ayman discusses how a potential for an autonomous reactor may be slowed due to regulation requirements. He also explains how his work is proving to regulators that smaller reactors are inherently safer than large reactors.)
Q. With all of these feedback loops, do we even need to control a nuclear reactor?
A. Researchers are working on designing autonomous reactors. They are theoretically possible and most likely to be developed first in small reactors. This is because the small reactors have different safety scenarios and consequences from the large reactors. When considering safety, the delayed release of energy, or decay heat, must also be considered. Decay heat is the heat left over after a reactor is shut down and is usually a few percent of the power a reactor generates. In large reactors, this percentage could be high enough to melt structural systems, requiring additional safety measures to be put in place. This risk does not exist in smaller reactors because the decay heat is low. Demonstrating this low risk could lead to reduced regulation. While this is supported, the change in regulation is taking a while to occur. This might be because of the potential to impact a populated area, triggering the industry to be overly careful. Ayman’s work shows that research reactors under a certain power level are inherently safe, meaning regulation may become less strict in the future. Ayman sees nuclear energy as a necessity for human progress. He finds it exciting because there are still things to be discovered, creating a great area of study for students. Ayman believes the future of nuclear is bright.
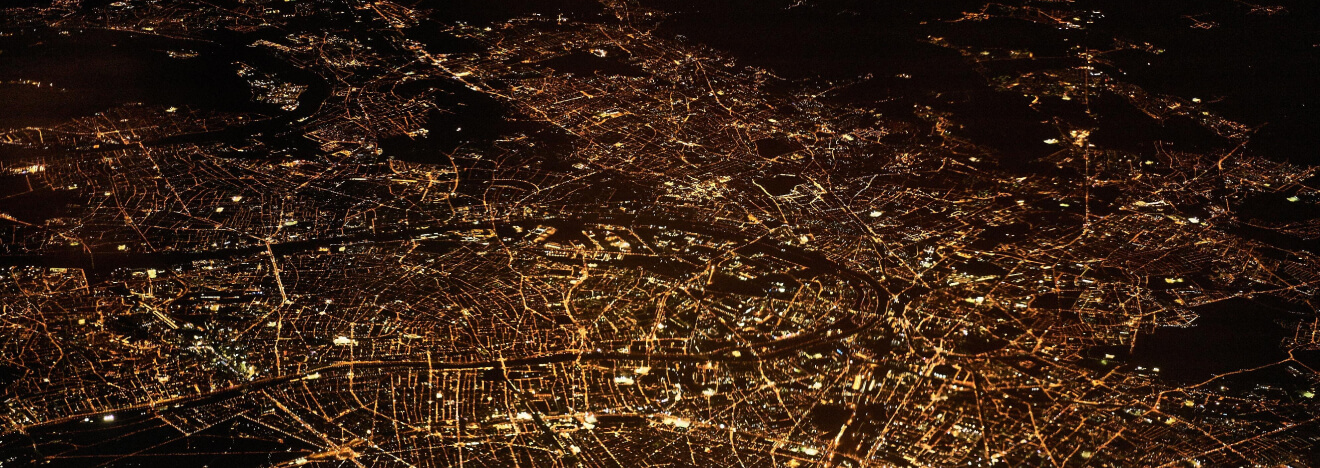