TITANS OF NUCLEAR
A podcast featuring interviews with experts across technology, industry, economics, policy and more.
Latest Episode

1) The beginning of Brian’s career and his time in the United States Navy as a diver, as well as what drew him to engineering and nuclear
2) Brian’s initial journey to Oregon State and all of the research projects he’s had a hand in since then
3) Fostering a passion for nuclear in the next generation of nuclear engineers and why the researchers as just as important as the research itself
4) What challenges and successes the nuclear industry will face in the coming years and how to form your individual opinion on nuclear energy
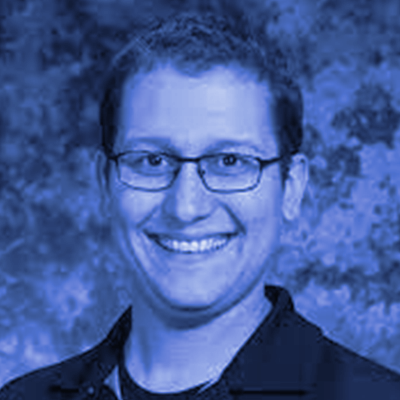
1) Michael Reichenberger’s entrance into the nuclear industry as a mechanical engineer
2) How fission detectors measure neutrons in nuclear reactors
3) The design, development, and deployment of Micro-Pocket Fission Detectors in a test reactor
4) Advantages of the Advanced Test Reactor for nuclear fuels and materials testing
Naomi Senehi: How did you enter the nuclear space?
Michael Reichenberger: Michael Reichenberger grew up in Kansas, where nuclear provides a significant amount of power to the grid, but was unaware of the impact of nuclear energy on his community. Reichenberger was drawn to study mechanical engineering at Kansas State University and fell in love with nuclear when he was required to take an introduction to nuclear engineering course. He spent all of his electives in the nuclear program and stayed at the university to pursue graduate studies focused on radiation detection, specifically micro-pocket fission detectors. Reichenberger was attracted to nuclear engineering due to its base in the logical sequence of physics. Nuclear engineers usually have a deliverable to apply the physics to, while nuclear physicists are more interested in understanding what is happening and why.
Q2
Naomi Senehi: What is a micro-pocket fission detector?
Michael Reichenberger: Michael Reichenberger’s graduate dissertation focused on micro-pocket fission detectors (MPFD’s), which are fission chambers that detect neutrons. A converter material that fissions, such as uranium, absorbs a neutron and splits into two parts which have a lot of energy. These parts go in two different directions and the energetic fission fragments creates ionization as it passes through a gas. This can be measured by applying an electric bias across the gas. MPFD’s are special because they can be put inside the core of a nuclear reactor without affecting the rest of the reactor. The smallest MPFD Reichenberger made had a chamber that was a third of a millimeter in diameter and was designed to go inside a flux wire cord in a test reactor in New York. It was challenging to push the wires inside the tube, so Reichenberger had the idea to braid the six wires together, which stiffened them successfully.
Q3
Naomi Senehi: What was the main premise of your PhD program and what did you uncover?
Michael Reichenberger: The title of Michael Reichenerger’s PhD dissertation was “The Design, Development, and Deployment of the Micro-Pocket Fission Detector”. The design phase goes back to basic physics to design the system to conduct a measurement. The development phase looks at how to actually build the detector using and sourcing materials that could be used at a nuclear reactor. The deployment phase involves putting the MPFD in the Trigger Reactor at Kansas State University and took real-time measurements at different axial locations in the reactor and during pulses. A nuclear reactor pulse is when the control rod is pneumatically ejected, allowing the reactor to go prompt supercritical and increase power exponentially. As reactor power increases, the temperature of the fuel increases and its strong negative temperature coefficient drives the temperature down, bringing the power down with it. Since this spike happens in a matter of milliseconds, most conventional tracking methods were not adequate to measure the neutrons.
Q4
Naomi Senehi: What advice would you give to students pursuing a PhD in the nuclear space?
Michael Reichenberger: Michael Reichenberger credited involvement in non-research activities and programs in helping him be successful in graduate school, by allowing him to network outside his core group and giving him another outlet. However, Reichenberger also recognized the power of saying “No” in order to identify your priorities and focus on the research when needed. Reichenberger now works at the Idaho National Laboratory as the Technical Lead. The Advanced Test Reactor (ATR) is the premiere irradiation facility for nuclear fuels and materials testing in the world which can sustain long cycles and high flux levels with large irradiation ports. The ATR has 45 different irradiation ports and nine different locations that can simultaneously be at different flux levels. The Radiations Metrics Laboratory (RML) supports ATR by conducting routine gamma spectroscopy measurement, measuring stack gas, and checking samples for unexpected activation. The RML also deploys and measures the radiation dosimeters that go into the core. Dosimeters are deployed throughout the core with cobalt and nickel wires, which become radioactive; after the cycle, the radioactivity of the wires are measure to determine how many neutrons they were exposed to.
Q5
Naomi Senehi: How can you use what you studied in your nuclear PhD in your role at Idaho National Lab?
Michael Reichenberger: Michael Reichenberger works at the Idaho National Laboratory (INL) as the Technical Lead. The value of real-time sensors is maximized in test reactors that have lots of power fluctuations, instead of commercial plants which have a steadier power output. However, the research and data collected at the test reactors translate directly into improvements for the commercial industry because research can move quickly. The current development cycle for a new fuel is 20 years due to testing and validation that must be completed to qualify the fuel, so there is ample room for improvement. Thermocouples have a large overhead to deploy in a test reactor so they are not always included in experiments. If there is an opportunity to unify the system and measurement by internalizing it to the unit, it could take some of the project-specific engineering for thermocouple deployment. The Advanced Gas Reactor at INL completed an experiment in which a gas lead was piped out into a measurement bay that goes to where the fuel is being irradiated. If there is a fuel failure, the fission product gases can be monitored. Innovation on how sensors are used can improve the data collected and used for commercial development.
Q6
Naomi Senehi: What are some of the new instrument types coming from the lab to be used commercially?
Michael Reichenberger: Since there is not much new nuclear construction in the U.S. at this time, Michael Reichenberger’s current instrumentation research is not ready to be commercialized yet. Sensors used in research reactors are more technically complicated and are innovative in the way commercial reactors are designed and build. However, these same sensors are not necessarily incorporated and used in commercial reactors. Data from research reactor sensors are used in commercial development, but commercial reactors primarily use sensors for operation and service. Having an integrated neutron sensor incorporated into the fuel levels could potentially provide burnup calculations, which may allow the current fleet to have longer fuel cycles or more efficient fuel usage. Instrumentation will be essential for the operation of advanced reactor designs, such as molten salt reactors, since there are currently no standard methods to conduct neutron methods in these environments. The nuclear industry will be sustained by maintaining the current fleet, which can be achieved by developing new fuels, and by developing reactor technologies. Idaho National Lab has a unified mission which empowers the team to work together and value each member of the team.
Q7
Naomi Senehi: What do you hope for the future of nuclear?
Michael Reichenberger: Michael Reichenberger is encouraged by continued development of integrated energy systems and people’s understanding the nuclear energy needs to be a part of the grid. Nuclear is essential to climate change. Reichenberger advocates for maintaining and extending the life of the current fleet of nuclear reactors, even as the next generation of technology is in development. The nuclear industry is very young, but people in the field have a vision for what is possible with nuclear energy.
7-10 Bullets
- Michael Reichenberger’s entrance into the nuclear industry as a mechanical engineer - How fission detectors measure neutrons in nuclear reactors - Reichenberger’s design, development, and deployment of Micro-Pocket Fission Detectors in a test reactor - The impact on nuclear reactor power and temperature when it goes prompt supercritical - Advantages of the Advanced Test Reactor for nuclear fuels and materials testing - How the Radiations Metrics Laboratory supports the Idaho National Lab by measuring irradiation - Benefits of real-time sensors in test reactors and how they impact development of commercial reactors - Potential value of real-time sensors in the operation of advanced reactor designs.
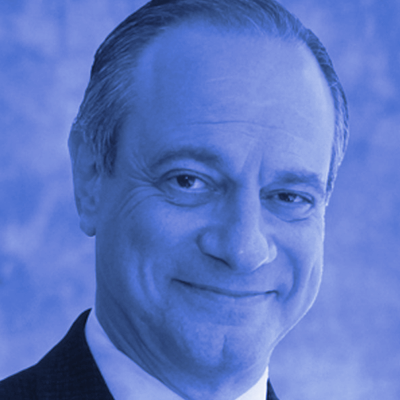
1) Neal’s role at the Nuclear Energy Institute and what nuclear energy has to offer to society
2) The importance of having advocates for nuclear energy that communicate the benefits of nuclear energy
3) How early education about nuclear power can influence public perception about the industry and its technology
4) A call to action for cooperation between generations to work towards a future without global warming through the use of nuclear technologies
1 - 03:40
Naomi Senehi: What is your background and what was your path to nuclear?
Neal Cohen: After acquiring a Masters degree in public policy at UC Berkeley, Neal Cohen went to Washington D.C. where he worked for a government commission focused on figuring out the relationships between the state, local, and federal governments. Cohen proceeded to Capitol Hill and worked with a new member of Congress on how to turn a superfund (hazardous waste) site into a useable space. For the next 30 years, Cohen worked for a consulting firm where one of the clients was the Nuclear Energy Institute (NEI), where he learned all of the benefits of nuclear energy that he was previously unaware of growing up in a very liberal democratic household. NEI was searching for somebody that didn't know anything about nuclear to run the campaigns to convert people from the political establishment into supporters of the industry, and reached out to Cohen who accepted the job offer. Neal Cohen advocates for representatives of the industry to analyze nuclear energy from an objective standpoint, independent of the engineering mindset that had previously dominated the conversations. Cohen emphasizes the importance of using language that resonates with the target group of people and provokes them to take action. Climate, clean air, national security, electrification, labor, and community are all problems nuclear can solve. The public’s fears need to be overcome by educating the population about nuclear energy as a technology that can save the planet.
2 - 11:23
Naomi Senehi: How do you, as a converted supporter of nuclear energy, convince people that nuclear isn't as bad as people believe?
Neal Cohen: Neal Cohen recognizes challenges in converting the public to be supporters of nuclear due to existing myths and fears that are overpowering the nuclear industry. A united and redundant message can impact public perception when supported and promoted by advocates for nuclear. Industry leaders with technical knowledge and a passion for nuclear can be the spokespeople so that senators, congressman, and state representatives become messengers support nuclear and take action. In order to support the future of nuclear energy, plants that are in jeopardy need to be saved and the infrastructure and workforce preserved. The battle is ensuring that nuclear is part of a broad portfolio of energy products. If nuclear power goes away, there are not enough energy sources to fill the void. Cohen looks to the Federal Energy Regulatory Commission (FERC) and PJM (a regional transmission organization) to push the consideration of externalities on energy prices. Without consideration of externalities, nuclear is always more expensive than natural gas. U.S. politicians need to promote the ability to export U.S. technology abroad so the U.S. is a world leader in the nuclear industry.
3 - 16:20
Naomi Senehi: How long do we have to convince people to get on board with nuclear?
Neal Cohen: Neal Cohen recognizes that the industry and country allowed nuclear to be something the public should be fearful of, stalling development and production which is needed now to produce clean energy. NuScale is learning from the past mistakes of nuclear in order to offer something better in the future and UAMPS (Utah Associated Municipal Power Systems) has committed to purchasing clean energy from NuScale. The Idaho National Labs has committed to siting the technology. Cohen fears that the U.S. is not keeping pace with what is required for technology and nuclear needs to be at the forefront of reversing climate change. A political consensus supporting clean energy needs to be formed to allow conversation about nuclear. The life of the existing nuclear plants can be extended for another 20 years with the use of new technologies, such as 3D printing and artificial intelligence. The grid needs smaller reactors that are more malleable and able to be constructed off-site and moved in, in order to bring power to new locations. The emergency zone around small modular reactors (SMR’s) allows these sites to be located closer to populations. Nuclear plants need to be efficient and run with well-trained employees.
4 - 22:59
Naomi Senehi: Why should we care about climate change and how does nuclear help?
Neal Cohen: Neal Cohen imagines a future planet filled with rising waters, conflicting economies, and displaced populations if climate change is ignored. Nuclear energy currently carries 55% of the clean energy in the U.S., while wind and solar only make up about 20% each. When there is no wind or sun to provide power, the renewables are backed up by fossil fuels, specifically natural gas. Nuclear is a 24 hour clean energy source and is essential for reducing carbon emissions. Education about nuclear energy should be implemented at all levels of schooling. This generation sees technology as a remedy to solve problems, and may not see nuclear as a bad thing, but don’t necessarily understand the core principles behind it. When technology leaders like Bill Gates step up to support nuclear, it impacts public perception in a positive way. Nuclear power plants are technically proficient and committed to a safety culture.
5 - 31:57
Naomi Senehi: What goals does the Nuclear Energy Institute have for nuclear energy on the power grid?
Neal Cohen: Neal Cohen sees the development of nuclear energy limited by regulators and politicians, who control design and construction. Nuclear energy, as an industry that reduces carbon, needs to be valued and needs to be competitive. Cohen advocates for the nuclear industry providing consistent power at 20% of the grid and ensuring it gets the recognition it deserves. This support opens up nuclear to further development. Cohen is working towards a better society so his children to see a future without a threat of climate change. Multiple generations need to collaborate to solve problems around climate, air quality, and jobs.
- Neal Cohen’s role at the Nuclear Energy Institute and what nuclear energy has to offer to society - The importance of having advocates for nuclear energy that communicate the benefits of nuclear energy - How to overcome the general public’s concerns about nuclear power through education - New technologies being used in old power plants to improve efficiency, production, and safety - The economics of exporting nuclear power and why the United States wants to lead this movement - The importance of established nuclear energy as a base load power source to support renewable energy - How early education about nuclear power can influence public perception about the industry and its technology - A call to action for cooperation between generations to work towards a future without global warming through the use of nuclear technologies.
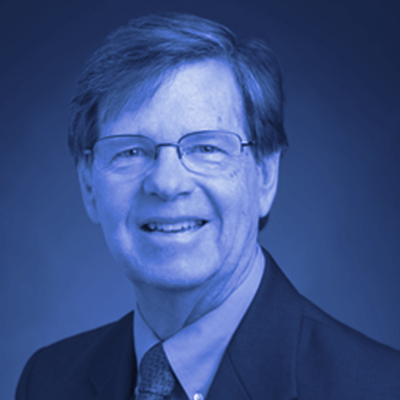
1) Technical challenges of scaling up high temperature nuclear power plant designs
2) How nuclear reactor designs plan for long-term material degradation in critical components
3) Modern methods of analyzing effects of radiation on metal alloys
4) How today’s nuclear industry is reinvesting in nuclear-related education and research
1) 00:36
Bret Kugelmass: Where did you grow up?
James Stubbins: James Stubbins grew up in Berkeley, California, when his father worked at Lawrence Berkeley Lab (LBL) as a research scientist, and then later, Cincinnati, Ohio when his father took a faculty position at the University of Cincinnati. Stubbins completed his bachelor’s degree in nuclear engineering at the University of Michigan and, due to Stubbins’ likelihood to get drafted during the war, had trouble finding work. Stubbins started grad school in Cincinnati and ended up not getting drafted. After graduation, Stubbins spent a year and half at a German nuclear research center, Forschungszentrum Karlsruhe, which later became Karlsruhe Institute of Technology, and then worked on nuclear materials at the University of Oxford and the Harwall Lab in the United Kingdom. Stubbins then moved on to General Electric where he worked on high temperature gas-cooled reactor technology.
2) 06:07
Bret Kugelmass: What is the relationship between the commercial sector, the nuclear industry, and the government?
James Stubbins: James Stubbins’ program at GE was purely research-based, at a time in which the Energy Research and Development Administration (ERDA) managed nuclear research while the Nuclear Regulatory Commission (NRC) regulated commercial nuclear power. ERDA was one of the organizations that later formed the Department of Energy. General Atomics developed the Fort Saint Vrain Reactor, a gas-cooled reactor in Colorado. The design was based on the Peach Bottom Reactor, but to a scale ten times larger, which created many unintended design challenges. Higher flow rates and a much larger core caused the core to shake, requiring a stabilization girdle. Since the reactor was built in Colorado, where there are no rivers to use for heat sync, a high temperature reactor which exchanges waste heat to air could be used. In nuclear plant turbines, the heat from the reactor increases the pressure of the gas, allowing it to be run through a turbine to extract energy. Fort Saint Vrain was running a steam cycle with the goal of using it for an efficient energy conversion cycle, such as from coal or iron ore.
3) 12:59
Bret Kugelmass: Why didn’t high temperature nuclear reactors become more popular earlier on?
James Stubbins: James Stubbins, who specializes in material science, sees the limit of very high temperature systems is the life of the materials in the components. High oxidation environments put an oxide scale on the surface, protecting against further corrosion and acts as a thermal barrier, but requires the parts and components to be replaced or rebuilt after experiencing creep. In a gas-cooled reactor, any oxygen in the system burns the carbon out of the core, so helium is used instead to protect the turbine blades from corrosion. A closed cycle system uses a gas as a heat transfer medium such as helium, which can withstand high temperatures. Hydrocarbons from oils in the system, like lubricated parts in the turbines, and there is always some air ingress. The challenge for these future plants is that carbon does not form a film on metal, but instead diffuses into the metal and changes the properties.
4) 17:37
Bret Kugelmass: Does a new metal alloy have to be developed for turbine blades in high temperature gas-cooled nuclear reactors?
James Stubbins: James Stubbins visualizes the development of a new metal alloy for use in high temperature gas-cooled nuclear reactors as a evolutionary process, rather than inventing something new. Material scientists analyze existing materials, then use the results to develop an alloy that works for this purpose. Japan has completed the alloy development cycle for use at a temperature of 950 Celsius. There is nothing to prevent the carbonization in these systems, which limits the material life and material degradation must be planned into the design. Scientists are trying to determine the ideal temperature, but plant efficiency is lost as the system temperature is lowered. Gases are not a good temperature conversion medium due to their low heat capacity and low density, compared to water, liquid metal, or molten salt, therefore pressure must be increased to keep high temperatures. One project Stubbins worked on with the Atomic Energy Commission analyzed what materials could be used for high temperature nuclear-powered planes.
5) 27:20
Bret Kugelmass: What other materials work have you studied in the nuclear field?
James Stubbins: James Stubbins has performed a lot of research on in-reactor materials that see a lot of radiation, such as fuels. Cerium, CeO2, is an atom that look similar to Uranium, so radiation effects can be performed on the atom without having Uranium present and understanding how the atom would respond. Some radiation effects performed include heavy ions and neutrons. Stubbins has also analyzed material that has gone through the Advanced Test Reactor at Idaho National Laboratory and also, in the past, looked at materials irradiated at the Fast Flux Test Facility, which is now decommissioned. The effort is to extend the performance behavior of materials to higher temperatures, higher doses, or higher stresses. Smaller, more compact reactors may require all of these increases in stress on materials. Stubbins analyzes what happens inside the material, and is currently utilizing the Advanced Photon Source at Argonne National Lab, which utilizes high power x-rays, to watch materials break apart. This allows researchers to paint a cohesive picture of the material response.
6) 34:53
Bret Kugelmass: Is the alloy metal a mixture or is it like a molecule?
James Stubbins: James Stubbins studies the effects of irradiation on materials such as alloy metals. Alloy metals are like a mixture in which the atoms are next to each other without atom-to-atom bonding, but small clumps of elements may form. Most metals have a lattice structure, and when a neutron comes in, it knocks atom out of their arrangement. Very energetic neutrons can cause atoms to knock other atoms out of order in a chain reaction. There is some residual damage, but often the atoms can fall back into a vacant place and restore the structure. The number of times the atoms in the structure are knocked out of place is analyzed for types of metals and the radiation environment they are in. Pressure vessels are forged, produced a better quality product, and are very thick and must withstand high pressures. They are intended to last a long time, instead of being a disposable component.
7) 43:38
Bret Kugelmass: Did you come to the University of Illinois after working at G.E.?
James Stubbins: After working for G.E., James Stubbins joined the faculty at the University of Illinois Nuclear, Plasma, and Radiological Engineering department. Right after the Three Mile Island incident, many reactors had to go be modified due to upgraded safety analysis regulations and lots of non-destructive testing on welds and other materials. This time in the U.S. also had very high interest rates due to inflation, so the nuclear industry took out loans at rates between 15-20% to support these upgrades. In the 1990’s, Stubbins saw undergraduate student numbers at the University decrease sharply, but was able to maintain graduate student population with high quality research programs. The nuclear industry at the time did not invest in university nuclear studies, other than some fuel development, but schools had very low budgets and funding. Stubbins became department head in 1999 and has seen a reinvestment in universities and nuclear programs, allowing the undergraduate population to grow. The University currently has 16 faculty with diverse backgrounds, allowing students to get involved in fields such as medical imaging, plasma technology, and fusion research.
8) 53:43
Bret Kugelmass: What do you down the line for you and the world in the nuclear space?
James Stubbins: James Stubbins is hopeful for the materials development space in the future of nuclear technology. Materials scientists today have better facilities for analyzing how materials behave and can tell more about the interactions. Additive manufacturing is changing the way materials are formed, allowing different compositions in layers of a structure to meet different needs.
7-10 Bullets
- Technical challenges of scaling up high temperature nuclear power plant designs - The relationship between nuclear power generation efficiency and reaction temperature - The effects of oxidation and carbonization on turbine blades in different nuclear reactors - How nuclear reactor designs plan for long-term material degradation in critical components - Modern methods of analyzing effects of radiation on metal alloys - How the impacts of neutrons on metal lattice structures drive material design - Performance criteria for critical components of nuclear plant materials - How today’s nuclear industry is reinvesting in nuclear-related education and research.
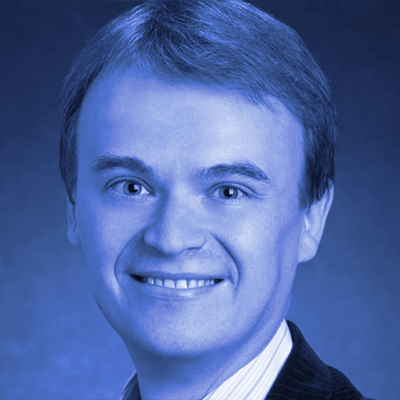
1) Why water is the best coolant in any reactor
2) Why molten salt reactors (MSRs) are a safeguard’s nightmare and how they differ from lightwater reactors
3) Why the Experimental Breeder Reactor II would be the best fuel reprocessing solution
4) Why the mass production of nuclear reactors is the only way to solve China’s energy demands
1- (0:22)
Interviewer name: Bret Kugelmass
Guest name: Tomasz Kozlowski
Title: Titans of Nuclear: Tomasz Kozlowski | Early Background in Nuclear and Path to Academia (Pt. 1)
Tomasz Kozlowski came to the US in 1993 and grew up in Northwestern Indiana. He took an early interest in science and technology, eventually attending Purdue University, the first school to accept him. Nuclear was intriguing to him, it was a mysterious attraction, and once he got started, he never looked back. Engineering and physics appealed to Tomasz, but not as much as nuclear did. Referencing the mysterious attraction of the field of “nuclear”, Bret commented, “What is happening inside an atom is an alien universe.”
After his Ph.D., Tomasz realized he had a preference for research. While looking for job opportunities, he found the Royal Institute of Technology, Nuclear Power Safety, in Stockholm, Sweden, and he served there from 2009 to 2011. Tomasz liked being a professor and mentoring students, it was personally satisfying to him.
After a few years and an assistant professorship, he came to the United States to further pursue a career in academia at the University of Illinois, where he is currently an Associate Professor of Nuclear, Plasma and Radiological Engineering.
2- (5:20)
Interviewer name: Bret Kugelmass
Guest name: Tomasz Kozlowski
Title: Titans of Nuclear: Tomasz Kozlowski | What is multiphysics? (Pt. 2)
Coolant is used to remove heat from nuclear systems. Tomasz specializes in multiphysics simulation for reactor safety. In his case, “multiphysics", means fluid flow and heat transfer combined, including analysis of neutronics and thermohydraulics coupled with the performance of the fuel. Tomasz solves simple analytical problems using metrics like "volumetric heat capacity", or the amount of energy required to raise the temperature of unit volume.
Water tends to be superior as a coolant since other industries and infrastructures are optimized to handle water. It is widely available and it’s easy to handle and transport.
However, due to certain constraints, different coolants, like sodium, lead, or water, may be “best” in different cases. A proper coolant may be determined based on the unique constraints and performance of the system.
3- (9:30)
Interviewer name: Bret Kugelmass
Guest name: Tomasz Kozlowski
Title: Titans of Nuclear: Tomasz Kozlowski | What are Molten Salt Reactors (Pt. 3)
Reactor physics is interesting and the academic field is robust, but molten salt reactors pose a unique challenge for contamination risk since the fuel is ground up into a fluid substrate. Enough released fission products from a system will make a nuclear plant shutdown due to radioactive contamination. Currently, it is necessary to contain radioactive material to a limited area.
In the example of automobile manufacturing in a large factory, it is difficult to absolutely prevent material leaks in an aging car engine. Likewise, absolute leak prevention in a nuclear power plant is nearly impossible. Some leaks in a nuclear reactor are expected and allowed, but the current question is, “How much leaking is acceptable?”
Theoretically, a simple cylindrical system can be hermetically sealed, but there are already small leaks in systems where fuel is hermetically sealed with high precision, so leakage prevention remains a hypothetical ideal rather than a standard of performance.
Molten salt nuclear systems present challenges. The biggest challenge from a regulatory standpoint is “safeguards,” or guaranteeing that no fuel is leaked, which is unrealistic but necessary to guarantee. Further, molten salt systems require volumetric calculations and exhibit a mechanism of extraction/insertion, which presents greater risks for tampering.
In Tomasz’s opinion, the best solution moving forward is “EBR-II”, or Experimental Breeder Reactor-II. It’s a sodium-cooled fast reactor at the Argonne National Laboratory National Reactor Testing Station in Idaho, USA, and it was shut down in 1994. EBR-II uses Uranium zirconium alloy rods as fuel, which can be electro-refined, melted, cast to alloy, and reused in a closed-loop system.
Sodium nuclear systems can be dangerous. When sodium leaks and comes into contact with water or air, it causes an explosion. Nevertheless, sodium is the best for reactors that require fuel reprocessing. However, if reprocessing is not required, water is the best coolant solution.
Molten salt reactors are not as prevalent because they are cost-prohibitive, even with recycled fuel, the cost of the power plant may be multiple billions of dollars.
4- (20:09)
Interviewer name: Bret Kugelmass
Guest name: Tomasz Kozlowski
Title: Titans of Nuclear: Tomasz Kozlowski | Purpose-driven Nuclear Technologies (Pt. 4)
Bret has been exposed to many different opinions on which reactors are better, e.g., lead, sodium, light, heavy, solid, etc. Tomasz uses the example of a car to demonstrate that nuclear reactors, like cars, have different designs based on their purpose. The best choice for a particular nuclear system is “purpose driven”, i.e., it depends on the purpose for the reactor and there are four different purposes a nuclear reactor may have, #1) electricity production, #2) heat production, #3) transmuting waste, or #4) breeding new fuel. For example, a light-water reactor is best for electricity production, gas or molten salt reactors are best for heat production, while fast reactors (lead or sodium) are best for waste transmuting and fuel breeding.
Bret asked “Why we aren’t building smaller light-water reactors or a new nuclear product altogether? Why haven’t companies in the oil industry developed nuclear solutions? Energy companies like Shell, BP, and Exxon are in the right industry and have the supply chain and resources to explore nuclear products.
Bret and Tomasz discuss the XKCD comic that displays the temperature of the earth over the last 20,000 years. Comic link: https://xkcd.com/1732/. Relative to changes that took thousands of years, major changes in climate have occurred in recent history.
5- (33:40)
Interviewer name: Bret Kugelmass
Guest name: Tomasz Kozlowski
Title: Titans of Nuclear: Tomasz Kozlowski | Renewable energy vs. Nuclear and Core Safety Analysis (Pt. 5)
How does Tomasz advise his students in order to help solve the problem of climate change?
Tomasz says that he tries not to give them his opinions, allowing them to investigate what they believe in. However, he is an advocate for large-scale nuclear energy production in contrast to other forms of “renewable energy” as alternatives to nuclear. Tomasz and Bret discuss confirmation biases and preconceived notions, and how they play a role in our own beliefs.
Tomasz’s current focus is core safety analysis, where he works to get the simulations to be as high-fidelity as possible. One key problem he is trying to solve is fuel failure mechanisms, specifically, in predicting when the cladding exterior around the fuel will crack and fail. Tomasz runs simulations at different cladding stress levels, including microcracks, grain morphology, and exposure to heat but these simulations do not always match experiments. The standard zirconium-cladding fuel rod in a light-water reactor lasts about six years, at which point, its ability to maintain production is mostly diminished.
6- (42:00)
Interviewer name: Bret Kugelmass
Guest name: Tomasz Kozlowski
Title: Titans of Nuclear: Tomasz Kozlowski | Simple Energy Production with the Light-water Reactor (Pt. 6)
Nuclear is easy to control and to construct, it does not harm the environment, and scalable mass production is possible. Even though nuclear energy is much simpler, cleaner, and less destructive than coal, there hasn’t been an intellectual drive towards 100% nuclear energy in past administrations of the US government.
China is relying more on nuclear and could be one of the countries to use 100% nuclear energy in the future. The Chinese will meet supply chain constraints before demand throttling.
Are we too timid to build enough nuclear power plants, similar to the scale of manufacturing automobiles? We tend to grow accustomed to the environment we’re used to and we must challenge our existing paradigms if full-scale nuclear is to become a reality. Mass production of nuclear reactors might be the only answer to clean energy and the reversal of climate change.
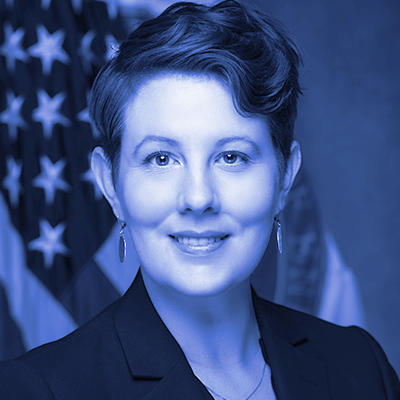
1) What a nuclear fuel cycle is and how it is analyzed
2) The connection between high enrichment nuclear fuel and inherent safety features
3) Computational tools for analyzing the nuclear fuel life cycle and transfer methods
4) Long term safety considerations for nuclear waste disposal of different isotopes
1) 00:56
Bret Kugelmass: How did you enter the nuclear space?
Katy Huff: Katy Huff grew up in rural Texas and was inspired by her mother, a mechanical engineer for GE in power systems, to pursue engineering. Huff spent the last two years of high school at the Texas Academy of Math and Science on the campus of the University of North Texas in Denton, Texas. For her undergraduate degree in physics, Katy Huff attended the University of Chicago, with a specific interest in its history in nuclear energy. As a graduate student at the University of Wisconsin, Huff worked on fuel cycle analysis, which looks at the life cycle of nuclear fuel from the moment it is dug out of the ground, how it is used in the reactor, and what is done with spent fuel.
2) 07:49
Bret Kugelmass: What do you know about the history of nuclear fuel fabrication?
Katy Huff: Katy Huff teaches fuel cycle analysis at the University of Illinois. In the past, nuclear fuel was enriched with gaseous diffusion, then technology transitioned to centrifugal diffusion, with the possibility of using laser isotope enrichment in the future. These processes separate Uranium-235, the fissionable atom, from Uranium-238, which can be fissionable; enrichment is increasing the ratio of Uranium-235 to Uranium-238. The biggest impact on fuel price trends is whether there is a surplus of enriched uranium. There are different levels of enrichment. Three to five percent enriched is typical light water level enrichment. Under 20% is still low enriched uranium, but reactors tend not to use this level of enrichment, with the exception of new reactor technologies in development. This power density is beneficial in smaller reactors and where high heat capacity plants. High enrichment fuel allows passive safety impacts related to fuel temperature feedback, which is a design aspect that allows a reactor to react to rises in temperature by reducing power levels, giving the plant an inherent safety feature that is not dependent on an operator.
3) 14:10
Bret Kugelmass: What are the drivers of negative temperature coefficients?
Katy Huff: Katy Huff analyzes the connection between advanced fuels and inherent safety features in newer nuclear reactors. Expansion, which decreases the probability of interaction, and doppler effect, which is a broadening of the range of energies when neutrons strike an atom, are two drivers of negative temperature coefficients in a nuclear reactor. Some advanced fuels have other passive safety features. New solid fuel shapes have altered heat transfer and how fuel melts by redirecting cooling in a spiralized way. New liquid fuels have heat transfer benefits which also allows fuel to expand quickly and provide negative temperature coefficients. In a typical light water reactor, heat in the fuel pellets travels to the edge of the pellets through conduction, the cladding is heated by the boundary condition on the fuel, and eventually transferred to the cooling system. Rapid heat transfer to the coolant allows stable fuel temperatures, reducing the risk of melting.
4) 22:04
Bret Kugelmass: Does the high temperature gradient between the fuel pellets and the cladding and the small distance create a problem?
Katy Huff: Katy Huff teaches methods and reasoning of improving the efficiency of heat transfer in a nuclear reactor system. Newton’s Law of Cooling says the higher the temperature gradient, the faster the heat will move. With advanced fuels that can reach higher temperatures, the higher temperature gradient allows a higher heat transfer behavior, which contributes to the ability to move the heat out of the fuel and into the coolant. It is easier to select a fuel for a reactor design than to design a reactor around an advanced fuel, since there are so many factors that affect design combinations. In academia, many computational tools are available to explore transfer methods, such as neutron transport, thermal hydraulics, and coupled multiphysics. Katy Huff’s group works with the Idaho National Labs by adding applications to model and simulate advanced reactors that don’t have appropriate computational tools yet. These tools assist students in implementing new methods and continuously programming to analyze reactor physics and options for design and technology in a reactor. The University of Illinois at Urbana-Champaign is home to Blue Water, the largest and fastest university supercomputer which allows Huff and her team to run simulations, but it not able to run all simulations due to security regulations.
5) 29:48
Bret Kugelmass: What research have you done on the back end of the nuclear fuel cycle?
Katy Huff: Katy Huff is interested in reprocessing strategies and disposal assessments in relation to the back end of the nuclear fuel cycle. A significant portion of Huff’s research has been related to simulating how reprocessing might work in the long term timeframe. Huff employs a fuel cycle code from the University of Wisconsin, used in her dissertation, which is an agent-based simulator for the nuclear fuel cycle. This program can assess which type of materials leave a nuclear reactor and with four different processes, what materials result. Reprocessing is when fuel, which is at the end of its usable life, is chemically dissolved to extract uranium, and possibly plutonium. Reprocessing is expensive, but some countries like France, have committed to the process with the belief that the benefits are greater than the costs. Dependent on the values of a nation, a country could incorporate externalities into economics through taxes, incentives, or national infrastructure.
6) 35:54
Bret Kugelmass: What are fission products?
Katy Huff: Katy Huff researches disposal assessments, and risks and benefits of methods and limitations. Some isotopes are of special interest to scientists due to their mobility in an environment, such as cesium or iodine, which basically have no solubility limit in water. However, these isotopes are particularly damaging to people and the environment, and solubility is more of a risk than a benefit due to long term storage and risks of entering water supply through accidental drilling. Katy Huff believes in continuing to hold the nuclear industry to a high safety and environmental standard, even if it is a higher standard than other energy sources. The economics of externalities can fix a market, such as carbon tax which would allow nuclear to compete with other technologies that do not produce energy without carbon emissions. While nationwide or international carbon credits or taxes do not yet exist, some states, like Illinois, have ensured that clean energy is being rewarded.
7) 44:45
Bret Kugelmass: What topics should the next generation of nuclear engineers be thinking about and focusing on?
Katy Huff: In a future of science and computationally enable lifestyles, Katy Huff believes in keeping the nuclear industry at the forefront of computing development. Nuclear energy and nuclear engineering, especially reactor physics, have been at the forefront of what algorithms are created. All of the supercomputers and large scale calculating have been created to complete nuclear energy calculations.
7-10 Bullets
- What is a nuclear fuel cycle and how is it analyzed? - How a surplus of enriched uranium impacts nuclear fuel prices - The connection between high enrichment nuclear fuel and inherent safety features - How negative temperature coefficients affect nuclear heat transfer - Computational tools for analyzing the nuclear fuel life cycle and transfer methods - Costs and benefits of nuclear waste reprocessing and how nations manage it - Long term safety considerations for nuclear waste disposal of different isotopes - Competitive advantages of nuclear energy and its impact on computational science.
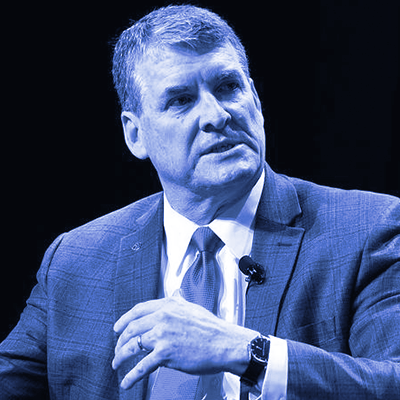
1) The impact of constructability in innovative nuclear reactor designs
2) How to turn nuclear design mistakes into lessons learned for the future
3) Differences between nuclear industry visions and realistic expectations for production
4) Opportunity cost benefits of nuclear energy that provide an all-around energy solution
1 - 02:02
Q: Where did you grow up?
A: Bill Fox grew up in Lancaster, South Carolina with his nine brothers and sisters, going on to received a degree in Civil Engineering from the University of South Carolina. When Bill Fox entered the nuclear industry in the late 1970’s, the U.S. had more than 70 large nuclear reactors under construction. Fox hired on with Duke Power Company, now Duke Energy, which was a utility able to provide their own design engineering and construction team with a highly developed supply chain. Duke Energy bought reactor technology and built a plant around it. This system would probably not work today due to challenges arising from plants getting canceled, due to being taxed on safety, the ongoing energy crisis, and escalation. Thousands of people lost jobs and supply chain went down. Bill Fox designed facilities from a structural and seismicity point of view, but early in his career, discovered that some of his innovative designs were not effective due to a lack of constructability. Fox now aims to teach and mentor design teams that an engineer’s goal should be to product an operational plant end product by designing to construct, instead of constructing to the design.
2 - 09:46
Q: How many new nuclear companies are starting with the construction site and ending with the reactor, instead of starting with the reactor and ending with the construction site?
A: Bill Fox admits to some scars from trying out new technologies in designing reactors for construction, but values the lessons learned from not doing everything right all the time. Small modular reactors (SMR’s) are sometimes advertised as being as simple as driving onto site and plugging in to start power generation, but it is a much more complicated process. Designers are good at the scientific part of the design, but the system that needs to be built around the science is more of a challenge. Designers must know what the plant needs to look at at the end of the project before groundbreaking in order to be successful. However, cost is sometimes advertised as very competitive, but without knowledge of actual build material quantities. Bill Fox and his team developed an SMR called mPower at Babcock & Wilcox (B&W). Just before starting work at B&W, Bill Fox was project director for the V.C. Summer nuclear project and developed the project site from the ground up for five to six years. His challenges in that process and understanding of the end product led him to an opportunity to get involved with new technology at B&W.
3 - 14:57
Q: What were the challenges did you learn the most from on the V.C. Summer project?
A: Bill Fox served as project director at the V.C. Summer project and recognized there was too much focus on the license as the end game, as opposed to the end game being an operating project. The economics to make a project successful is a challenge and since project success is determined before a shovel is put in the ground, planning is essential. V.C. Summer did not get all the details right in the design process and was the first time a project operated under a Part 52 license, which is a combined operating license. Under Part 52, any change during construction required the license to be opened back up and reconsidered. After V.C. Summer, Bill Fox joined Babcock & Wilcox (B&W) to develop the mPower small modular reactor (SMR).
4 - 18:58
Q: What happened to the mPower small modular reactor?
A: Bill Fox joined Babcock & Wilcox (B&W) to develop the mPower small modular reactor (SMR). A significant challenge with reactor development is securing funding. Money must be spent up front to develop details in order to get cost and schedule predictable, but funding might be provided until cost and schedule is predictable. Federal government funding assisted B&W on mPower. Hundreds of millions of dollars were invested in the design in order to created predictable cost and schedule to attract investors. One benefit of working in Canada is that the environmental industry, regulation, and government are all pointing in the same direction. Canada has an environment that bring revenue back if the plant is built, as opposed to environments where reactors must “bid into” the grid. Opportunity cost benefits, such as climate change, energy independence, national security, fuel independence and diversity, grid stability, and medical isotopes are not measured and instead, the focus is on supporting the cheapest source of energy.
5 - 24:05
Q: How did you end up at SNC?
A: Bill Fox currently serves as the Executive Vice-President for SNC-Lavalin. Fox always had two drivers in career: being able to make a difference and participating in continuous learning. Fox took time off after running the federal and commercial operations group at BWXT and started his own consulting business working on small EPC projects. Fox got a call from SNC to get back into the nuclear business, including refurbishing 10 reactors across Canada and new build opportunities. Bill Fox accepted the position and is passionate about building Canadian technology to a point at which it can be exported. SNC is the largest engineering and construction company in Canada, with a large global presence. The company has experience with CANDU technology and can support different SMR technologies and take deployment models to the field.
6 - 28:46
Q: Is there a generic balance of plant design that could be used across multiple small modular reactor companies?
A: Bill Fox sees an industry vision to have a generic balance of plant design that could be used across multiple small modular reactors (SMR’s), but it is only a vision and not a reality. Lots of intangibles, such as transmission lines, water sources, the environment for cooling water, closed loop, and cooling towers create one-offs in the plant. Enveloping site conditions are created to account for seismicity, wind and thermal, and dispersion effects with design inputs. However, when site conditions are enveloped for the worst conditions, overdesign and conservatism causes price to go up. Cost and price predictability must be obtained before execution. Change kills projects, including changes in regulation. The regulator needs to understands the industry and the projects; Bill Fox sees the Canadian Nuclear Safety Commission (NSC) doing a good job of that. A combination of private capital with government support is needed to provide risk support. Developing technology costs hundreds of million dollars before construction, and with more than twenty potential designs, the development costs go way up.
7 - 35:23
Q: Where do you think nuclear technology should narrow its focus?
A: Bill Fox recognizes that Canada needs a lot of small reactors in rural areas, along with mid-scale reactors to plug into the grid. The customer cares about having energy at hand, and not so much about what specific technology is used. The design must be infiltrated into the supply chain for critical equipment and components. Industry should self-select which nuclear reactors go into production. SMR’s design-to-operation takes too long to be practical, and the industry must be realistic about the timeframe for small reactor implementation, especially considering that new technologies may come down the pipeline to fill the energy gap.
8 - 41:15
Q: Why is nuclear energy important to the future?
A: Bill Fox is concerned about the environment and energy in the future. Energy is the lifeblood of any economy, and if it starts to be depleted or the energy mix challenged, the economy will follow. People don’t know where the energy comes from that powers activities at night and in their cities. Small modular reactors (SMR’s) are also great vehicles to provide desalination services to clean water and heat homes, which has worldwide implications.

1) How Paula Maguire worked her way up to become a training manager for Ontario Power Generation
2) How collaboration between utility stations can impact a plant’s operations and the nuclear industry as a whole
3) The importance of leadership in a highly technical field such as nuclear energy
4) The impact of transparency and open communication in the nuclear industry
1 - 00:26
Q: How did you enter the nuclear industry?
A: Paula Maguire worked her way up through the utility industry to eventually become the Training Manager for Ontario Power Generation, with a focus on the nuclear utilities. Maguire originally started work at a roller shade manufacturing facility out of school, covering all aspects of the business at some point. She joined Ontario Hydro to work in the mailroom, but had the opportunity to take courses and went into both accounting and energy management. The company paid for Maguire to return to school to pursue Building Environmental Sciences, and Ontario Hydro evolved into Ontario Power Generation, or OPG, and Maguire got involved with the training organization.
2 - 03:31
Q: What is a training organization and what role does it play at a nuclear utility?
A: Paul Maguire’s involvement in the training organization at Ontario Power Generation (OPG) had a direct impact of the day-to-day operations at a nuclear station. The training program taught employees the knowledge and skills to do the job, but also focused on lessons learned from the performance of stations. Human performance errors sometimes caused events that impacted the function of the station, and the training program communicated these mistakes to employees to prevent further incidents. The training managers looked for trends in types of events and analyzed how training positively or negatively influenced performance. The training organization offered training for both staff and craft, with a heavy emphasis on leadership. The Senior Nuclear Plant Manager program brought in leaders from all over the world and put them through one week in the classroom learning about leadership and one week at a utility to survey the leadership team at the station. Training like this is vital to the nuclear industry, since a mistake at one utility affects all utilities and the industry as a whole, which encourages lots of sharing between groups.
3 - 09:35
Q: How did your responsibilities change from when you first entered the training aspect of the organization through your career?
A: Paula Maguire first entered into the utility training program to develop coursework for a new software that was being implemented, and eventually went on to become the PIckering Site Training Manager before retiring. As training manager, Maguire was responsible for the certifications and qualifications of everyone working at the station. All employees at the utility have a training program based on their position and require continuing education. There is also opportunities to train up to the next level position. Paula Maguire stresses the importance and impact of helping employees understand the “why” of training and the value added for individuals and for the company, in order to achieve top results as a team. Paula Maguire now serves on an audit team with the International Atomic Energy Association, tasked with completing operational safety analysis review team assessments. The IAEA has created guidelines for how nuclear utilities are supposed to operate, and the purpose of the audit is to verify compliance with the guidelines. The three week assessments look at the utility training organization, leadership at the station, corrective action plan, and how expectations are being met with concrete examples.
4 - 14:47
Q: What are some cultural differences between Eastern Europe and Canada?
A: Paula Maguire has witnessed a care and attitude towards nuclear plants in Eastern Europe that is similar to that in Canada. She observed that other countries may be less open about mistakes, perhaps due to pride or shame, whereas in the U.S. or Canada, everyone is transparent about mistakes made and lessons learned to prevent repeat events. Maguire encourages individuals to provide honest feedback regarding how the organization is operating, without fear or reservation. If a utility has a certain weakness, Maguire’s team provides references to utilities that have strengths that could be beneficial, to be an example for successful program development and implementation.
5 - 20:09
Q: How do you think companies should conduct themselves going forward to get the most out of their workforce?
A: Paula Maguire values transparency as the number one way to maximize the return from the workforce at the nuclear utilities. Open and honest communication lets employees know and understand more about the reason “why”, leading to more personal investment in the program and the company. Maguire also recognizes that leaders and managers should coach and develop their staff, while letting the technical staff to excel in their work.
Top Bullets
- How Paula Maguire worked her way up to become a training manager for Ontario Power Generation - Purpose and structure of a utility training organization - How collaboration between utility stations can impact a plant’s operations and the nuclear industry as a whole - How to inspire your utility workforce and maximize their value at the station - Importance of leadership in a highly technical field such as nuclear energy - Purpose of operational safety analyses and how they differ across the globe - Impact of transparency and open communication in the nuclear industry.
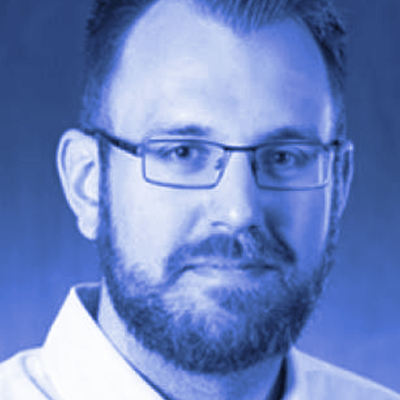
1) How Sean Donnelly was inspired by the dynamic and unique challenges of the nuclear industry
2) The important role Kinectrics has played in helping to develop nuclear technology
3) Why placing U-Batteries at Chalk River and Urenco would be the best models for what this technology can do for costs and power generation
4) Nuclear's pivotal role in the future of clean energy
At this time we are still producing show notes for this episode. Please check back again at a future date.
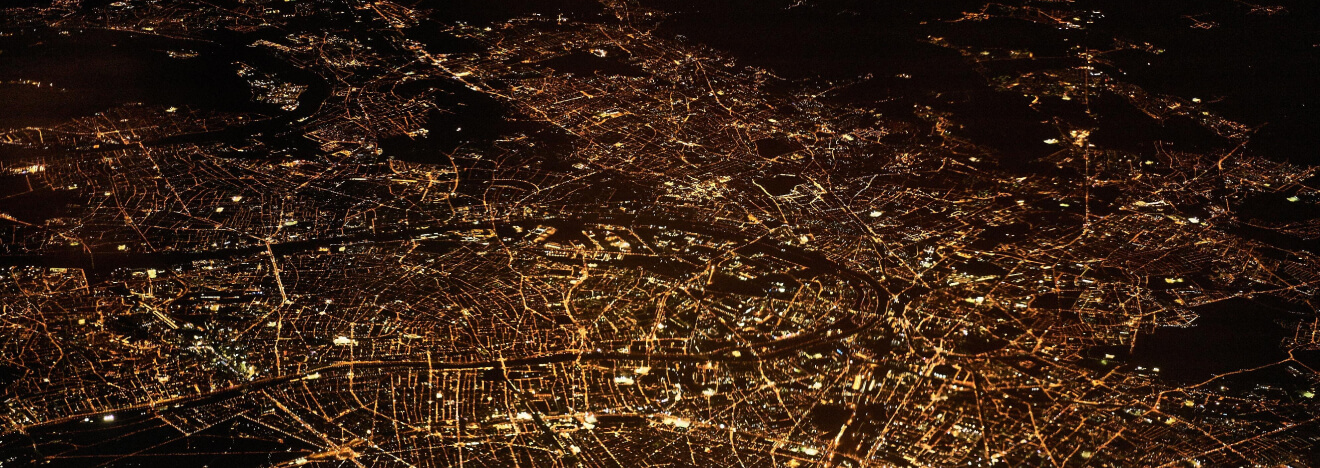
Sign up for our newsletter
No results found
Please try different keywords