Ep 378: Tammy Ma - Lead, Inertial Fusion Initiative, Lawrence Livermore National Lab
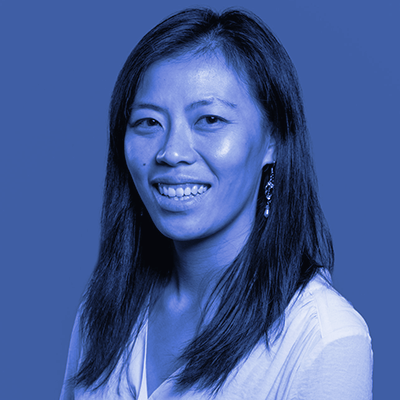
Show notes
Bret Kugelmass [00:00:59] Okay, so we're here today on Titans of Nuclear with Tammy Ma, who is the Lead of the Inertial Fusion Energy Initiative at Lawrence Livermore National Labs. It's so great to have you on. Thank you so much for joining us, Tammy.
Tammy Ma [00:01:10] Thank you so much, Bret. It's an honor and a pleasure.
Bret Kugelmass [00:01:13] Yeah, well obviously, some great news has come from your facility over these last few weeks and I'd love to get into that. But before we do, we'd love to learn about you as a person. So start off by telling us, where did you grow up?
Tammy Ma [00:01:26] Oh, I actually grew up here in the Bay Area in northern California, in Fremont actually, which is just over the hill from the lab. It's about 20 miles away. So in high school, I actually got to participate in some of the lab's outreach events. They used to do something called... We actually still do something called Science on Saturday, where scientists give public lectures about their work. And I do remember hearing a talk about the National Ignition Facility and huge lasers and fusion. And at the time, I didn't understand a whole lot, but I got inspired by the idea of doing like big science, working on big teams, and knew I wanted to come back to the lab, come work at the lab someday. And so I've been very lucky that that's the way it's worked out. My family's still here in the Bay Area, so I get to live close by to them.
Bret Kugelmass [00:02:20] Who are some of the early influences in your life that got you excited about science and engineering and technology?
Tammy Ma [00:02:25] Oh, absolutely my parents. My dad was an electrical engineer. My mom was not a scientist. But they just would get so excited about science breakthroughs and take us to science museums. They loved science and so that actually trickled down. Both my brother and I are now physicists, actually.
Bret Kugelmass [00:02:45] Oh, that's so cool. That's something I always like to hone in on because like for some people it's kind of unfortunate. Like, if you don't have an engineer in the family, sometimes you don't even know what engineering is. And I think we definitely have to increase people's exposure. I mean, I think they've done a good job getting STEM more on the agenda in general, but it often is that some of the best engineers had family members who were engineers that coached and guided and pushed them from a very early age.
Tammy Ma [00:03:10] Yeah, I mean, I completely agree. I had no idea what research meant. You know, it was a combination things, parents, but also like I had some really great science teachers in middle school and high school, and that makes all the difference too.
Bret Kugelmass [00:03:27] Did you do competitions like Science Olympiads and stuff like that?
Tammy Ma [00:03:28] I did not. I did not. It wasn't big at my school at the time.
Bret Kugelmass [00:03:35] Now your school, Freemont, that's where the big automotive manufacturing facility was, right? Did that trickle into the school system and stuff?
Tammy Ma [00:03:41] Yeah, so actually my dad worked at the Toyota plant, which has now turned into that Tesla plant. I think it's not just that factory, it's just being in Silicon Valley also. There's a lot of educated people, there's always a lot of new breakthroughs and excitement around tech and science, and I think that seeped into my bones personally.
Bret Kugelmass [00:04:04] Sure, sure. Okay, great. And then so, walk us through your academic experience a little bit more as well. Where'd you go to school, grad school, all that stuff?
Tammy Ma [00:04:12] Sure. I did my undergraduate at Caltech, the California Institute of Technology.
Bret Kugelmass [00:04:17] Quite a school.
Tammy Ma [00:04:18] It was hard. I have to say I cried a lot. But I picked to go there because I wanted to be an aerospace engineer. And Caltech happens to run and operate the Jet Propulsion Laboratory, which is the national laboratory in charge of all non-manned missions. And so for me, that was just like a dream, right? And so I did major in aerospace and then decided to go to graduate school and I thought I was going to do work in plasma thrusters because I had done a little bit of summer research as an undergrad. I was looking around for advisors, ended up going into plasma physics. And with plasma physics, there's all different applications of plasma physics in different regimes, and I ended up going into fusion. And so I did my graduate school at UC San Diego. So you can see there's a pattern. I've stayed in California pretty much my entire life.
Bret Kugelmass [00:05:20] And did they have experimental facilities there or is it more theoretical at this point in your experience?
Tammy Ma [00:05:26] Well, so we at San Diego did not have big lasers. There were smaller labs, of course, but not big lasers.
Bret Kugelmass [00:05:37] So still experimental and not theoretical physics.
Tammy Ma [00:05:38] Experimental stuff. But what I ended up doing was actually traveling around during my graduate years to different laser facilities, including here at Livermore, to do experiments.
Bret Kugelmass [00:05:46] How did that happen? Did the school sponsor that? Was it a specific program, or did you just make it happen on your own?
Tammy Ma [00:05:51] It's, you know, the professor gets his funding grants from NSF or Department of Energy or whatever, and that pays for the execution of these experiments. And you can have money to actually send students to travel around to do that work.
Bret Kugelmass [00:06:07] And what was your thesis specifically?
Tammy Ma [00:06:10] It was on... I think the title of it was "Transport of Energetic Electrons for Fast Ignition Fusion." What that means is you take a laser, and if you shoot, say a solid, the laser is so intense that it liberates the electrons from the rest of the atom. Basically, you generate... and those electrons get accelerated, get very high energy, and you can use that as a heater for different fusion mechanisms.
Bret Kugelmass [00:06:41] Cool. And when the electrons emit, is it directional or do you just very quickly control the direction with like a magnetic field?
Tammy Ma [00:06:54] Well, no, that's a great question. They're somewhat directional. They also have like a spectrum of energy. So it's not one single energy. So the name of the game is exactly that. How do you control what energy comes out? How do you control where those electrons go? And so work on that is ongoing. It's a very difficult problem.
Bret Kugelmass [00:07:16] Can you help describe, since you probably spent a lot of time thinking about this, can you help me create a a visual understanding or an analogy for what an electron actually is? Protons and neutrons, I got it. I think of like a small circle closely packed together with mass. What is an electron?
Tammy Ma [00:07:38] So an electron is a negatively charged particle.
Bret Kugelmass [00:07:42] And it's a particle? It's actually a particle. Are we sure?
Tammy Ma [00:07:45] Yes. Oh, am I sure? Are we getting theoretical here?
Bret Kugelmass [00:07:49] Because it's just so small, and it doesn't have much mass. I mean, how do we really know?
Tammy Ma [00:07:53] No, no, it's definitely a particle. And so, what's interesting about the electron is, like you said, the protons and neutrons are packed close together, creating the nucleus. The electrons, you know, kind of circle around the nucleus and they contain a ton of energy and they actually form a little more of a cloud rather than having discrete places that they always are. But what's interesting about electrons is they are despertized in their energy in the sense that once you liberate...It takes a very definite amount of energy to pull an electron off, and then you very specifically might emit X-rays or other signatures that that happened. And then each electron in its particular place also has a very specific energy. And so you can do very, very cool physics with that if you can record as you're pulling electrons off or adding electrons back in, what's actually going on in the core of this atom.
Bret Kugelmass [00:09:00] And okay, one more question on electrons for now. This idea of them existing in this cloud, that's more of like a probabilistic interpretation of their position. How do we describe their motion though? Is it an orbit or are they just like randomly appearing in different places at different times?
Tammy Ma [00:09:20] I mean, we still call it an orbit, but it's not... You know, in your high school textbook, we draw these little circles. Rather than a 2D circle, it's actually a 3D sphere that electrons are moving in. And so it's still accurate to call it an orbital. Where that electron actually resides is a function of the coulomb energy, the attraction and repulsion forces that sets exactly kind of where the electron is with respect to the nucleus.
Bret Kugelmass [00:09:59] But it is moving within that orbital? Or it is appearing and being in places within that orbital?
Tammy Ma [00:10:06] It is moving in that orbital.
Bret Kugelmass [00:10:06] It is moving. Okay, okay. So there is motion. Okay, cool. Great. All right. That was just a personal curiosity satisfied. All right. Now, let's continue on to what happened post-grad school.
Tammy Ma [00:10:16] Sure. So I did both my master's and my Ph.D. at UC San Diego. Again, like I said, I was traveling around. I think towards the end, the last two-ish years of my graduate school, I actually moved up here to Livermore to work more closely with the scientists here and the facilities we had on site. And then I graduated in 2010, which was fortuitous because the National Ignition Facility, the world's largest and most energetic laser, was just coming online. We had just completed construction of the facility, we were just starting experiments. We needed experimentalists to develop and do experiments there.
Bret Kugelmass [00:10:59] And what was the purpose? When Congress was first asked for money for the National Ignition Facility, what was the intended purpose of it?
Tammy Ma [00:11:07] Multiple folds, certainly. But the main purpose is what we call science-based stockpile stewardship. This is almost a little bit of a change of topic, so bear with me. What science-based stockpile stewardship means is that, in 1992, we put a moratorium on underground explosive nuclear testing. This is the Comprehensive Test Ban Treaty, and it is something that we, the U.S., and many countries around the world agreed to. We're not going to test anymore. However, we, the U.S., and many other countries still have nuclear arsenals. And what that means is that nuclear weapons that we had previously built are buried under the ground. Okay, cool. So they're there, but we can't explode one every once in a while to see if they still work, so how do we ensure that that stockpile stays safe, secure and effective, you know, if God forbid, we did need to use it? But more importantly, to use it as a deterrent. Their usage as a warning other countries completely disappears if we don't know if they work or not. And so the NIF was constructed to feed into this because with the NIF, we can generate conditions, the very high temperatures, densities, pressures similar to what you have inside nuclear weapons at a very tiny scale. But to be clear, we are not generating, we're not building new nuclear weapons. We are not detonating bombs. We can just study similar physics on the NIF. And that same physics we can then feed into our simulation computer codes and make sure that our codes are accurate against the physics. And those same codes are what we use to ensure that the stockpile is safe and effective.
Bret Kugelmass [00:13:09] So let me try to paraphrase for our audience. In order to not have to do experimental testing, we want to do this analytical testing on a computer. But these computer programs, especially physics simulators, are really quite complex. Like, they are hard even for the best coders and best physicists to compile these computer programs. And so, often we have to benchmark the results against something in reality to fine tune, you know, twist this dial a little bit, change that algorithm a little bit. And so rather than testing them against blowing something up, we're testing them against something that is still in the same realm of physics, but is wholly different. And that's what the NIF facility is.
Tammy Ma [00:13:48] That is exactly right. Thank you, that was a great explanation.
Bret Kugelmass [00:13:53] No, it came from you, I was just trying to learn it.
Tammy Ma [00:13:55] Yeah, that is exactly it. And then the other point is to train up people like me and my colleagues who are the stewards of this physics. You know, how do we make sure that we have those exquisite skills that also tend to be a little bit unique and make sure that we have that continuing. And that also is a deterrence to our adversaries because it's a very visible signal of the expertise that we have in this arena.
Bret Kugelmass [00:14:30] Yeah. To keep smart people, you need to give them toys to play with.
Tammy Ma [00:14:33] Yeah, that's right. Yeah.
Bret Kugelmass [00:14:34] Okay, great. So now, teach me about some of these experiments that happened throughout your career, and what culminated in this momentous occasion?
Tammy Ma [00:14:46] Yeah, absolutely. So the NIF runs 24/7. It is, like I mentioned before, the world's largest, most energetic laser. It is actually 192 separate lasers. Each one alone is one of the most energetic. So you can imagine, when we're combining 192 of them, like you said, Bret, it's a pretty cool toy, right? And the building that it's housed in is the size of three football fields, side by side, ten stories tall. So it's enormous. And the reason the building is so big is we have to house thousands, thousands of optics for each of these lasers. And those optics are what we use to amplify the lasers up in energy. So we are not talking about your typical little laser pointer, we are talking about "ginormous" lasers. What we do then is we take all of this laser energy and we're going to shine it back down on a tiny target. And this target contains our fusion fuel, which for us is deuterium and tritium, these isotopes of hydrogen. And the target sits in a tiny capsule about two millimeters in diameter. And that capsule sits in what we call a hohlraum, it's a little canister, something that is made of something that's high Z, so high up the periodic table.
Bret Kugelmass [00:16:16] High Z, that described like the weight of...
Tammy Ma [00:16:20] High Z, exactly. So gold, for us, or depleted uranium. The idea is when you use these high Z materials, we're going to take the laser energy, shine it on the hohlraum, and then the hohlraum generates X-rays. And so, back to our earlier conversation about electrons, when you use a high Z material, something far up the periodic table, it means there's more electrons. More electrons you can liberate and therefore you get more X-rays.
Bret Kugelmass [00:16:48] But the X-rays are not a particle.
Tammy Ma [00:16:49] The X-rays are not. No, that's right. So the X-rays then kind of fill this little canister and we generate an X-ray oven. So like your oven at home, where the idea there is you're trying to get uniform heating all in a small space so that you cook, for us, we're using X-rays to cook our little capsule. And the capsule, that little shell that I talked about, which is typically plastic or some sort of carbon, it ablates. So it blows off super fast. And it's a rocket-like reaction. So by conservation of momentum, you're blowing up the shell super fast. The rest of it wants to compress in really quickly, and then that's how we compress the fusion fuel up to high density. It will also get incredibly hot. And then if it's hot enough, dense enough, and you hold it just long enough together, then you get fusion ignition, so more energy out than you put in. And that is the name of the game, and that's what we achieved last December.
Bret Kugelmass [00:17:55] And just so our audience can visualize like the physical parameters of the system, so we started off with this three football field, ten stories high, houses all of this laser equipment. The final layer of optics of all of these lasers, how close do they sit to the target?
Tammy Ma [00:18:16] Yeah, great question. So the lasers are going to be bouncing back and forth in this "ginormous" facility, getting amplified up, then they all get pointed around this target chamber. So it's this enormous vacuum chamber that is spherical, it's a huge ball and it is 10 meters or 30 feet in diameter. And it's held at vacuum because our lasers need to propagate in vacuum, otherwise they would get disturbed, basically. And this target chamber basically weighs, it's made out of aluminum, 130,000 pounds. It was so big we actually had to put the target chamber in first and build the facility around it, to give you a sense of size.
Bret Kugelmass [00:19:09] That's awesome. Okay, and then how is the pellet suspended? I'm assuming it's in the middle of this chamber, this vacuum chamber? How is it suspended in the middle?
Tammy Ma [00:19:19] I love how you're breaking this down. The target sits on this enormous boom arm that pushes in and out of the target chamber. So I said before that the chamber is 30 feet in diameter. In order to hold the target in the middle, that boom has to be at least 15 feet, the radius. And so it's this arm that is incredibly stable. Can you imagine like holding your arm out and you have to hold that target to a precision of about 20 microns, so that's about 1/10th of a human hair in precision?
Bret Kugelmass [00:19:55] Wow. Are you using a lot of like piezoelectrics at the very end to get that final positioning or something?
Tammy Ma [00:20:00] I don't actually even know. I think so, yeah. There's piezoelectrodes farther back too, like that hold the arm very stable. And then each laser, as it approaches the target chamber gets focused down. So each laser comes down to about 200 microns, so two human hair-ish size, pointed very specifically on a certain location on that target. And so not only is the NIF the biggest laser in the world, it's also the most precise laser in the world in many different ways.
Bret Kugelmass [00:20:40] Cool. And then does that boom arm interfere with the... because I assume... And I understand this is more like a test facility rather than a prototype of a commercial power production facility, but would that perhaps be an engineering complication? I assume you probably want to have as few materials as possible as close to the source of the reaction. How do you deal with extra materials in your neutron envelope?
Tammy Ma [00:21:18] For these particular experiments, you're exactly right. It's a scientific demonstration facility. The boom is what it is, and then there's different parts holding the target farther out. The target gets obliterated every shot. We make sure of that, in fact. You don't want chunks of debris actually falling back onto the laser and the optics.
Bret Kugelmass [00:21:41] How big is the target again?
Tammy Ma [00:21:43] The little sphere that I was talking about is two millimeters in diameter. The hohlraum that it sits in is about a centimeter in length and half a centimeter in diameter.
Bret Kugelmass [00:21:51] Oh, the hohlraum is that small. That's the thing that translates your laser energy into gamma energy. Or you said X-ray.
Tammy Ma [00:22:00] It is X-ray. So you can imagine that target being so tiny that the lasers have to be super precise so you can hit it, right? We have an analogy that the lasers are equivalent to a pitcher standing on a pitcher mound at Giants Stadium in San Francisco and then pitching a perfect straight down at Dodger Stadium in LA, to give you a sense of scale.
Bret Kugelmass [00:22:33] That so good, that's so good. Cool. And then that little pellet, what are the materials in that pellet again? Is it pure DT?
Tammy Ma [00:22:44] Yes. So it is deuterium-tritium. What we have to do is right before we push the target into the chamber, we have to fill that pellet with the DT fuel. And so there's actually a little straw that sticks in to the capsule. We feed liquid DT in at 50/50 concentration. What we actually have to do then is actually bring the temperature down and freeze that DT into an ice. So just like water ice, H2O ice, in this case, it's just DT ice.
Bret Kugelmass [00:23:24] And is that because in order to increase the probability of a reaction, you want the atom distance from one to another to be closer physically?
Tammy Ma [00:23:35] No, actually, it's not close enough at that point. It's actually because we need to pack enough fuel in there that you could have propagating...
Bret Kugelmass [00:23:47] The density of fuel.
Tammy Ma [00:23:47] Yeah, but there's also a trick. What we do is we hold them... We bring the temperature right down to around the triple point. And so actually there's a DT ice layer, but right in the center it is actually gas. And so gas is more compressible. But we need that ice layer to pack...
Bret Kugelmass [00:24:04] Triple point of hydrogen?
Tammy Ma [00:24:05] Of the deuterium and tritium fuel mixture.
Bret Kugelmass [00:24:08] Okay. Are there any other elements in there?
Tammy Ma [00:24:12] No.
Bret Kugelmass [00:24:13] But there kind of has to be some small percentage, right? Because your tritium has, what, like a two year half life or something? So, like, it can't be pure in the sense that from the time that you made it to the time you get it in there, there's got to be some other stuff mixed in, right?
Tammy Ma [00:24:27] So it's about, I think it's a 12.3 year half life. You get a little bit of decay, like you get beta decay, so you form other particles, but it's not other atoms. There are no other materials. It is pure deuterium and tritium. And then the capsule around it...
Bret Kugelmass [00:24:48] Oh right, because you're so low on the periodic table anyway, there's not much else that could become in your decay.
Tammy Ma [00:24:54] Yeah, that's actually right.
Bret Kugelmass [00:24:56] Yeah, I understand. Okay, and then what percentage of your mass actually gets converted in this reaction when you fire?
Tammy Ma [00:25:15] The amount of fusions that you have, because you're going to fuse deuterium-tritium, and in that reaction you generate a helium nucleus, what we call an alpha particle, and that energetic neutron. So the number of fusions that we actually create is directly related to the amount of energy you generate. For us, on that December shot where we got ignition, we actually burned through just 4% of the fuel. You can imagine if you could have a more efficient reaction and burn through much more of that, how much more energy would you generate.
Bret Kugelmass [00:25:52] Or could you repeat the laser shining process... I'm calling it shining, sorry, it's probably the wrong word... On that same pellet to eject, to like eek more out of it?
Tammy Ma [00:26:02] Oh, so you can definitely change the behavior of the laser. So for us, when we say we shine the laser on, it's not again, like your laser pointer at home, where you turn on and off. For us we can very carefully modulate the power output as a function of time. So we actually do little bumps up in laser energy. We turn it back down, little bump up, big rise up. And what that does actually is sends shockwaves, very precisely, due to compression.
Bret Kugelmass [00:26:33] And when you say it sends shockwaves, I'm thinking of like, you know, those funny experiments that you see where people like use sound waves to make like water move and you can create different... Is this essentially, is it that you are trying to have the shock waves intersect at a point?
Tammy Ma [00:26:52] Yeah, exactly. We're doing a spherical compression. So we're sending in spherical shocks to fully compress that capsule down.
Bret Kugelmass [00:27:01] Got it, got it. And it's that compression that increases the probability that your deuterium and your tritium will interact with each other. Or it's actually overcoming the coulomb energy?
Tammy Ma [00:27:16] Yeah, I would say we do the compression to get up to the densities and generate the heat that we need to overcome the coulomb repulsion between the deuterium.
Bret Kugelmass [00:27:28] So it's all about overcoming the coloumb energy.
Tammy Ma [00:27:30] Yeah, it is.
Bret Kugelmass [00:27:32] For DT, what is that? It's like 100 million degrees or something? What is it?
Tammy Ma [00:27:38] Well, it's a spectrum, right? It becomes more probable the hotter you get.
Bret Kugelmass [00:27:47] Where's the peak?
Tammy Ma [00:27:48] It's around 10 keV. So I think that's 100 million degrees Celsius if I'm doing the math right in my head.
Bret Kugelmass [00:27:57] Okay.
Tammy Ma [00:27:58] 10 keV is right though.
Bret Kugelmass [00:28:00] Cool. And then how do you measure again? How do you measure the energy output?
Tammy Ma [00:28:07] So the energy comes out in that energetic neutron that is generated in the fusion reaction.
Bret Kugelmass [00:28:14] And how many neutrons? I don't remember your initial mass, but you're getting 4% of it. So how many neutrons total are coming out in this event?
Tammy Ma [00:28:22] Oh, for that big ignition shot we had over 10^17th neutrons.
Bret Kugelmass [00:28:31] Cool.
Tammy Ma [00:28:32] That's a lot of zeroes, right?
Bret Kugelmass [00:28:33] And then you're able to count them by essentially having a detector over a certain area at a certain distance away, and then it's just, you just count?
Tammy Ma [00:28:39] Exactly, exactly. And we have more than one detector, certainly. They're arrayed all around the chamber. We know the solid angle that that detector is measuring over. We see the number of neutrons that arrive there. And then just like you said, we can then recalculate the total number and verify it among different...
Bret Kugelmass [00:28:56] Yeah, I was about to say. And what is the difference between... What is the sensitivity of any given one sensor? Do you have to do some math on top of multiple sensors to figure out what the right number is?
Tammy Ma [00:29:06] Yes. So we have 120 different instruments arrayed around the chamber. They're not all for measuring neutrons, but a good number are. The detectors, they measure neutrons of different energies as well. They're located at different places and in different distances from the center of the target chamber. And what you want to do is measure the neutrons coming at the detector right there and make sure that you are consistent across all the detectors. And of course, you're not going to get exactly the same number for all of them, but then you get an error bar on your measurement.
Tammy Ma [00:29:45] The other thing, one very accurate way that we also measure the neutrons is by activation. And so certain materials... It's as simple as just a little solid foil. It could be copper, it could be tantalum. They're arrayed all around the chamber and they get activated. When the neutron comes in, that material gets into an excited state, and then it has a half life of decay that you can measure very accurately over time, and then use that also as a detector. And those decays actually do take time though. Sometimes hours, sometimes weeks. And so that's why right after a shot we know something big happened, but it still takes time to verify the number. And as scientists, we want to be rigorous and make sure we also have good error bars and understand the uncertainty in the number that we quote.
Bret Kugelmass [00:30:39] And then, these neutrons, they're non-directional. Is it equal? Does a neutron emit in every direction with an equal probability?
Tammy Ma [00:30:52] For our fusion reactions, yes, because it is a thermal reaction. We just have a pellet that is spherical, that's filled with deuterium-tritium. And so the fusion should happen in a way that neutrons are omnidirectional in how they are emitted. However, they should be. Not always, because what happens is when you do that compression, you're not always perfectly symmetric and spherical, right? It's like compressing a balloon in between your fingers. It's really hard; anywhere it can, it's going to push back out, right? And for us, it's a similar thing. We have 192 lasers. It's still not, you know, enough. It's tough. It's really tough to squeeze something symmetrically. But that's important. It is a signature for us. We can measure the neutrons and we can see the asymmetry, and that's the signature for us where we might need to improve the implosion on the next experiment.
Bret Kugelmass [00:31:50] Cool. So cool. And then, how do you deal with neutron scattering, like as it intersects with that boom arm or other parts of your equipment? The neutron is going to, some are going to scatter, some are going get absorbed. How do you account for that? Or at least mathematically, how do you model or understand where they went before they hit your final detector?
Tammy Ma [00:32:11] Right, that's a great question. We can model, first off, with very good simulations. There are very good neutron particle scattering and computer simulations that you can model all of this and know kind of what to expect. And then we can also do test shots where we know we're emitting a certain number of neutrons. Let's see where they go. Make sure that our diagnostics are calibrated against things like scattering or the spectrum being a little bit different from what we expect. And so all of that is taken into account mathematically as we do the analysis.
Bret Kugelmass [00:32:53] And this goal of getting more energy out than you put in, how long has that been the goal of this particular apparatus? Or was it even? Or is this just a fun experiment on top of it?
Tammy Ma [00:33:09] It absolutely was a goal. The idea of getting more energy out than you put in through a fusion reaction with lasers has been going on for over 60 years now.
Bret Kugelmass [00:33:21] 60? Six zero?
Tammy Ma [00:33:23] Six zero, since the laser was invented. About two days afterwards, one of our former lab directors actually came up with the idea of, "Well, why don't we use lasers for fusion?" And so, here at Livermore, and we're not the only ones doing laser fusion, but here at Livermore, we've had a series of bigger and bigger, more energetic lasers. Now the NIF itself, we started construction in 1997, we completed in 2009, and so we've been doing experiments from then through to today, which is about 13 years. And yes, it's always been a goal because once you achieve ignition, you are in this different plasma regime where it's like you light a match, right, and that little flame propagates. For us, that propagating burn puts us in what we call the burning plasma regime, and you can generate huge amounts of what we call high yield, lots of neutrons that you can use for other experiments. And furthermore, it opens a path towards fusion energy as a viable energy source someday. Of course, if you can generate more energy than you put in, if you can turn that up even more, very easily you can see how maybe we can start feeding that out to the grid.
Bret Kugelmass [00:34:44] And then the key is how do you make it self-sustaining? Because you don't want to have to power up the laser every time for every shot, right?
Tammy Ma [00:34:51] No, we would power the laser up every shot. So, the NIF, like you alluded to earlier,Bret, is a scientific demonstration facility. We do an experiment once every 4 to 8 hours or so by design. And our experiments are very complex; everyone is a little bit different. In a fusion power plant, we would need to repeat the reaction about 10 times a second.
Bret Kugelmass [00:35:18] To create like what power level of thermal energy output?
Tammy Ma [00:35:23] The plans that we have now would be to generate a gigawatt type power plant similar to our coal powered plants of today.
Bret Kugelmass [00:35:34] So 10 shots a second can theoretically create a gigawatt or three gigawatts thermal?
Tammy Ma [00:35:44] Yeah, about a gigawatt, gigawatt electric. So there's still many, many challenges ahead. Not only would you have to do it 10 times a second, we would need quite a bit higher gain than we've demonstrated so far.
Bret Kugelmass [00:36:03] And how involved is your group with the effort to commercialize this technology for power production verses scientific achievement?
Tammy Ma [00:36:19] Yeah, not just scientific achievement, but also what we call the stockpile...
Bret Kugelmass [00:36:24] I should have started with that. Yes, yes, I didn't mean to...
Tammy Ma [00:36:33] I just started a new job, actually. And my job is the Lead of Inertial Fusion Energy Initiative here at Livermore, which is exactly that. You know, how do we take this and translate it into what could be a viable energy source?
Bret Kugelmass [00:36:48] And what was your job before? Right before?
Tammy Ma [00:36:50] And I'm still doing it. I run a scientific group using these very high intensity lasers to do, you know, cool physics with fusion physics, what we call high energy density experiments, shock physics, all kinds of things.
Bret Kugelmass [00:37:06] Okay, so now I want to ask the question more about you than about the science. How did you step into this role, both from a qualifications perspective, but also from an interest level perspective? What made you the perfect fit?
Tammy Ma [00:37:22] I had done experiments on the NIF for years and years in support of inertial confinement fusion, which is exactly... It could be exactly the approach that you would want to use for fusion energy. So right now, these different missions that you and I have talked about, Bret, stockpile stewardship, energy, even just some of the discovery side itself, are all aligned. It's exactly the same right now. And then there's different applications, like so many of our different technologies, right? We are at the point where if we want to go towards fusion energy, a lot of different technologies now need to be developed.
Bret Kugelmass [00:38:03] Like the engineering part, not just the...
Tammy Ma [00:38:04] Like the engineering, and that's going to be a hell of a job, exactly. And so, for me, I started getting more into policy work a few years ago. Going to D.C. And working with our program managers at DOE headquarters and talking to staffers on the Hill to both share the work that we do here on the NIF, communicate the science, which is always super important, but then to start laying the groundwork for the future. There were a lot of skeptics, but, you know, here at Livermore, here at NIF, we believed we would get ignition, it was going to be imminent. Then what's the next step? We have to be ready. And so starting a few years ago, I got very involved in that communication, working with the community to put together reports to Congress or to DOE to say, "Here are the challenges that we see in the next few years. This is where we would like to see investments to push the field forward." And so that's how I got more involved in really growing this new program, which turns out is more policy than it is technical, as I'm finding out.
Bret Kugelmass [00:39:27] Well, you seem quite capable of both. So how do you balance... Okay, this is more to like the social aspects of it than... How do you balance the excitement around the achievement with... One thing that I'm afraid of is... I mean, this is amazing in the sense that we got so much positive press. I mean, every newspaper reported on this. But then, the reality of the engineering challenges to turn this into a commercial power production facility could take how long? You fill in the blank for me.
Tammy Ma [00:40:11] Yeah. That's absolutely true. What we've accomplished on the NIF is a huge scientific breakthrough, no matter what. What we've been trying to do with our communications is be completely transparent and be very rigorous in how we talk about the science. We're not claiming that you could plug the NIF into the grid and pull energy out. We've been trying to articulate the many different challenges that are there, not just in the science and technology that still needs to be developed, but also the support that we need from government and private companies and all these different stakeholders and use that, in fact, as a way of of trying to communicate that we still need investment and we still need policy to be developed in a way that sustains this new technology. And then making sure also, for us, the U.S. is the leader in inertial confinement fusion. That's undisputed, right? Yes, we would love to have fusion energy available to everybody around the world because it's such a clean, abundant, wonderful source of energy if we can make it work, but the U.S. has the leadership now. We need to maintain that leadership, to capitalize on it, to make fusion energy work.
Tammy Ma [00:41:38] In the meantime, there are all these technology spinoffs. There's just overall scientific prowess we need to maintain. And so it doesn't benefit us to make promises that cannot be met. We need to articulate the challenge. We don't even have the staff right now, the workforce, to put multiple power plants on the grid any time soon. It takes years to build that up. For me personally, I find that inspiring. And we hope that also is the message that can get out to schoolchildren today. But at the same time, when the media gets excited, that's great too. But we do try to stress that it will take probably a few decades to make fusion energy something that is ready. But you know, that timeline very much, very, very much, depends on the actual investment and the will. If the U.S. makes a strong commitment now, we can move a whole lot faster.
Bret Kugelmass [00:42:57] Other than understanding the physics, which you guys have demonstrated incredible prowess at, what are those next areas of focus that will enable a commercialization of this technology? Is it a set of material science challenges for the enclosure? Is it a way to make the laser optics more efficient? What is the next set of technical challenges that you'd like to see investment in?
Tammy Ma [00:43:19] Yeah, absolutely. You totally hit the nail on the head. Materials is a big one. We need materials that can withstand huge fluxes of being irradiated or particled for what would be the chamber walls of the reactor. We need materials that are hardened. So, the optics that deliver the laser. You can hide a lot of those optics in other places and shield them. However, at some point, the laser needs to meet the target chamber and there has to be a final optic. How do you protect that? How do you prevent damage?
Bret Kugelmass [00:43:58] Especially with those damn omnidirectional neutrons.
Tammy Ma [00:44:00] Yeah, exactly. Those neutrons, they're tough, right? And so materials is the big one. And there's work that needs to be done with tritium processing and recycling.
Bret Kugelmass [00:44:20] Because you've got the fuel source.
Tammy Ma [00:44:20] The fuel source itself, right. So tritium is a limited resource. So in a fusion power plant... You asked a little bit earlier about how much of the fuel do we actually burn up. You do not burn up 100% of the fuel. It is really tough to burn all that fuel up before the pressures get so large, it just like re-expands. And so you would want to recapture any of the fuel that doesn't get used and repurpose it and recycle it and send it through the system again. So there's a decent amount of work that needs to get done there. It is not an easy problem because you are working with the tiniest atom, the smallest atom that we have on the periodic table. It seeps into everything. That's one of the challenges. There are challenges with automation and bringing in machine learning and AI. So automation, you would drop a pellet in 10 times a second, the lasers need to track it and shoot it. But things are moving so fast, you can't have human intervention, right? Everything needs to be controlled remotely. Also because it's a power plant where there's lots of energy flowing in all different kinds of directions.
Bret Kugelmass [00:45:44] You just brought up something. You used the words "drop it." And that does actually beg the question, given how precise you are already with your timing of the lasers, why hold the pellet instead of just dropping it and timing the lasers to meet? Because the speeds are relatively slow of it dropping and easy to calculate with gravity relative to your lasers. Why not just let it drop and hit it right when centered?
Tammy Ma [00:46:09] So in a power plant we would, but right now for the scientific facility, we're only doing a shot every couple of hours. And so it doesn't make sense at this point to add that additional level of uncertainty. But you're right, it would not be the most difficult subsystem that we need to bring up. There's work that needs to be done, but we've got that challenge down, I think.
Bret Kugelmass [00:46:32] Does this laser driven approach work with other fusion reactions other than DT?
Tammy Ma [00:46:44] Yes.
Bret Kugelmass [00:46:44] Specifically a neutronic reaction, would be my net follow on question.
Tammy Ma [00:46:49] There are certainly some private companies looking at the neutronic reactions. Anything that is... DT has the highest cross-section.
Bret Kugelmass [00:46:59] It's easy.
Tammy Ma [00:47:00] It is the easiest one. And it already took us 60 years to get it, right? But it is the easiest one. As you try to fuse other elements because they are bigger, heavier than hydrogen, you need to put more energy into the system to even start those fusion reactions. So it just adds an additional level of difficulty.
Bret Kugelmass [00:47:24] Yeah. I mean, where I was just thinking about it though, it's like now that you demonstrated the concept, rather than tackle the engineering... There are going to be a lot of engineering challenges dealing with neutrons. Now you've demonstrated the concept to instead work on the challenges, just get more energy into the system and not have to deal with those pesky neutrons, maybe that's an easier problem in the long run to solve.
Tammy Ma [00:47:50] Potentially, potentially. But there are a lot of trade offs that you have to kind of look at there.
Bret Kugelmass [00:48:01] Can you actually talk about a couple them? You don't have to go super deep, but I would love to hear how you think about the trade offs.
Tammy Ma [00:48:06] Yeah, sure. So for a couple of the candidates, you're probably thinking of D-helium3, can fuse, DD can fuse, deuterium-deuterium, or P-boron. So a proton to boron. With P-boron, you have to get... We said that the peak... Let's get nerdy here. So the peak of the DT cross-section, so where we get the most fusion happening... Or the probability of fusion is the highest for DT at around 10 keV. For P-boron, it's 100 keV and the cross-section there is still 1,000 or 10,000 times lower. So even if you can get up to 100 keV, even then, the probability of getting fusion to happen is much lower. So there is that complication.
Bret Kugelmass [00:49:09] People are trying to do P-boron reactions out there, right?
Tammy Ma [00:49:12] Absolutely. Yeah. And you can get P-boron to fuse, right? That's been demonstrated; you can make it happen. But it's just like us, until this big shot that we had in December... We had plenty of DT fusion, you just don't have... What you need in most cases is what we call propagating burn. You need to spark that and then get the burn to feed on itself. That's the only way that you can actually make the energetics, the equation work out to have more energy coming out than you put in.
Bret Kugelmass [00:49:46] Okay, so there is a bit of a chain reaction happening.
Tammy Ma [00:49:48] Yeah, there is a little bit of a chain reaction. That is true, yes.
Bret Kugelmass [00:49:57] Okay, cool. And then, maybe talk just a little bit more about how your program is going to interact with commercial efforts moving forward. Is it that they come to you guys for advice? Is it that they want to use your physical equipment for experiments? How do you do this? Do you call it technology transfer, or how do you do it?
Tammy Ma [00:50:23] Yes, it is tech transfer? Yes. It's a little bit all of the above. Right now, for the Department of Energy, public-private partnerships is a big thrust. Not just in fusion, but kind of across the board in all different technologies, particularly energy and climate security type research. And so right now our job as a national lab is to do exactly that -- technology transfer. So we are not a production facility. We typically do things once. After they get solved, they get passed over to either private companies or other institutions, commercialized. And so with these private companies, there's a number of different ways. We do collaborate with them; we share expertise. But there are also tools that we have built up over the decades, and these include the computer simulation tools and the facilities and then other component technologies that we might have here as well. And so, we sign contracts with some of these private companies where they contract us to help develop a specific thing they need, or we build up a collaboration with multiple private companies and we say, "Hey, guys, what do you all need in common?" What we call pre-competitive technologies, technologies that everybody will need at some point, those are the type of technologies we focus on at the national labs to develop and then everybody can use them equally.
Bret Kugelmass [00:52:01] Cool. Great. Okay, well, we're running low on time, though. You covered so much material in an hour, it was just amazing talking to you about this. But I'm going to give you the final word though. What else would you like our audience to think about, know about, understand, or you can even end just with what your hopes for the future are.
Tammy Ma [00:52:20] We're so hopeful about fusion and its potential. What we're trying to do now though is develop the fusion industry, the ecosystem, in a way that is equitable, diverse and just. And that means not just developing workforce that is diverse, but really thinking about now like, where would you site a nuclear fusion power plant? You know, they're safe; their footprint is no bigger than probably what a coal power plant would look like. So hopefully people are amenable to having that in their backyard, but we need to communicate out what those benefits are. And then the other way we're thinking about it, back to the equity thing is, where would you put these plants that are most beneficial to society? We don't want this to be an energy source that only benefits the rich, the privileged folks. It is something that could really be an enormous step change in lifting the standard of living of folks everywhere, especially in developing countries as well. I guess what I'm trying to get to is we need help not just on the scientific and technical side, but on the policy side, understanding what the user base is and working with communities and outreach. And so, we're just starting out now. It's a really exciting time, and we hope people will consider fusion as a career and join us in this challenge.
Bret Kugelmass [00:54:00] Tammy Ma, everybody.
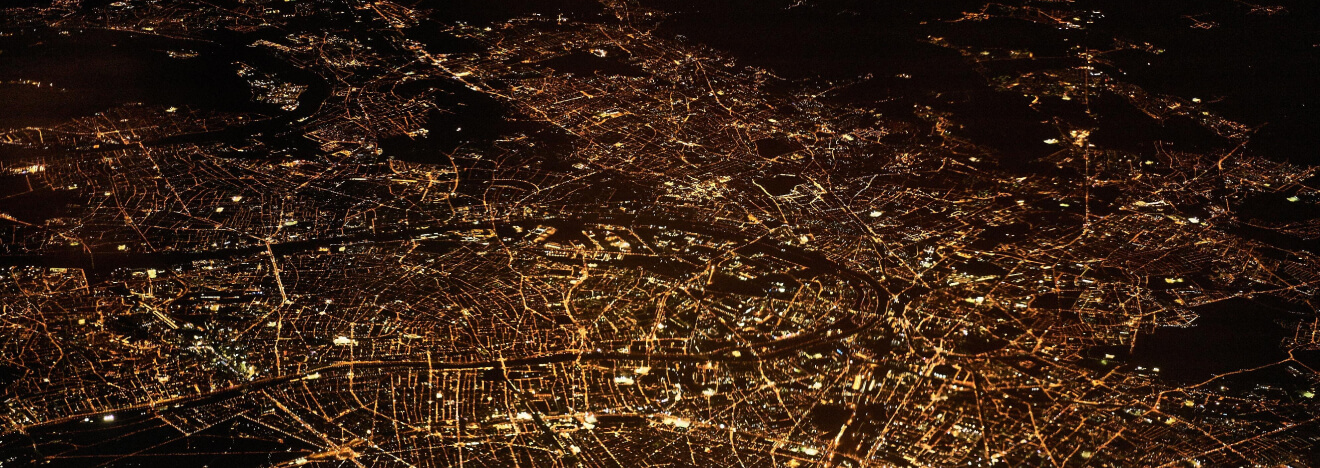