Ep 02: Per Peterson - Chief Nuclear Officer, Kairos Power
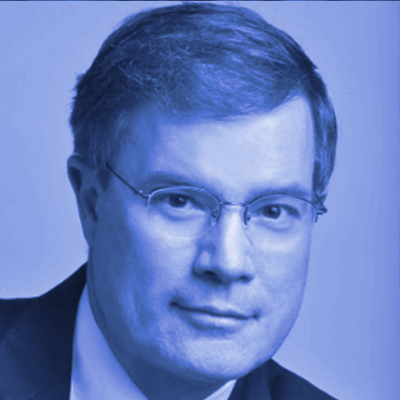
Show notes
Q1 - Entrance into Nuclear
Bret Kugelmass: How did you get into the nuclear field?
Per Peterson: Per Peterson’s background is in mechanical engineering, but he has always had a strong interest in energy and the environment. Looking at the most important problems for humanity to solve, the big coupling between what humans can do and the environment involves energy and agriculture. Peterson became interested in solving problems that are difficult to solve and realized that nuclear energy has enormous potential. The costs for the quantities of material required to build nuclear power plants don’t make sense, so there are significant problems around how we design and build these technologies. However, the cost of raw materials doesn’t matter for nuclear power because it is a small fraction of the total cost; the highest cost is the salaries of the workforce. The quantities of uranium that are available are enormous and the ability to use it more efficiently can be implemented. Thorium can also be used. It’s difficult to conceive a world in which uranium prices would be significant enough to matter in the generation of nuclear energy.
4:23 Q2 - Civil Nuclear Energy and Nonproliferation
Bret Kugelmass: What attracted you to the nuclear space and how did you come to your conclusions about material cost and energy?
Per Peterson: Per Peterson completed his graduate studies in mechanical engineering at UC-Berkeley and minored in energy and resources. During his Energy and Resources 200 class taught by John Holdren and Mark Christensen, Peterson was impressed by Holdren’s view on nuclear technology and how he ranked the different issues associated with it. Waste was ranked at the lowest point, safety of reactors and reprocessing facilities midway up, and nuclear nonproliferation was at the top of his list. This convinced Petersen that working in the nuclear field was very important. Regardless of whether we use nuclear power for civil purposes, humans need to remain competent about how we manage the military dimensions of the technology. International institutions have been developed to do that, and the Nuclear Nonproliferation Treaty and the International Atomic Energy Agency are impressive accomplishments in the global nuclear field. When you look at civil nuclear energy from the perspective of nonproliferation, the ability to have strong incentives to work in good faith and have peaceful use has brought broad compliance. Within the civil sector, materials can be segregated and handled in forms that are not directly usable in nuclear weapons, such as enriched uranium. In South Africa, which had a clandestine nuclear weapons program based on highly enriched uranium, the government decided divest itself and transfer materials to the civil sector when the apartheid was going to collapse. For about 25 years in the United States, half of the electricity in nuclear power plants was begin generated with uranium that had formerly been in Russian nuclear weapons. Nonproliferation safeguards, physical security, and cybersecurity and infrastructure can be designed to have synergies between these objectives.
9:21 Q3 – Spent Fuel Comparisons of Nuclear and Coal
Bret Kugelmass: Why is nuclear waste at the top of the public’s minds?
Per Peterson: Properly managed nuclear waste is easy to isolate from the environment. For the people that understand nuclear technology and have studied it, many do not consider spent fuel from reactors a waste. It is feasible to recycle, even though it is not economic right now. The current technology with water-cooled reactors is extremely expensive. Fuels for advanced reactors that use coolants other than water are much easier to fabricate from recycled materials. It is better for us to store spent fuel in the interim and wait to see significant deployment of these advanced reactors. Some people are opposed to nuclear energy for a variety of reasons and believe that nuclear waste disposal is dangerous. In 2015, nuclear power provided 11% of worldwide power generation, which offset combustion of a billion tons of coal. The total amount of spent fuel generated that year would fill an American football field to a depth of 1.3 meters. One billion tons of coal would have filled the same field to a depth of 230 kilometers. There is no practical way to burn a billion tons of coal and not discharge all of the waste into the atmosphere. A very small number of repositories would be needed for deep geologic isolation and the worst case is isolated contamination of groundwater, which has already been done pervasively with chemicals to a magnitude larger amount. The incremental public health impact would be very small.
15:13 Q4 - Geologic Disposal Capability in the U.S.
Bret Kugelmass: What is the industry consensus on the way to explain spent fuel disposal to the public?
Per Peterson: There is an obligation to move forward and develop geologic disposal capability. Of the different legacies passed on to the next children, nuclear waste has scientifically valid technical solutions and the amount of work to manage that waste is modest, while there is no plausible approach to get the carbon dioxide out of the air and the oceans. When you look at what is being done with fossil fuels, there are large scale risks of ecological disruption and it is difficult to rationalize worries about nuclear waste disposal. Nuclear waste is not preventing us from building nuclear plants. The Department of Energy (DOE) is required by law to write out a contract for any utility that wants to build a nuclear power plant to take full responsibility to manage the spent fuel that is generated. The amount of money that has already been paid for disposal may be sufficient that there may be no need to ever charge utilities again in the future for managing spent fuel. Utilities are also required to have decommissioning funds and put into highly secure investments. Price-Anderson caps the total private liability to $10 billion if there is an accident and the U.S. federal government picks up the rest of the cost.
19:35 Q5 – Merging Old and New Reactor Technologies
Bret Kugelmass: Do we continue with the old technology with incremental improvements or do we start developing a new, advanced technology?
Per Peterson: Per Peterson supports both incremental improvements of existing technology and development of new, advanced technology. Governments generally are not great at making technology selection decisions. There is a lot of logic supporting keeping existing plants running, since they are fully depreciated and they have low production costs, unless there are markets with large amounts of overproduction on an intermittent basis which costs the plants money on negative electricity prices. Renewables will continue to deliver electricity to the grid, even when the losses are negative, because they can still collect a subsidy. In Germany, the decision has been to continue running coal plants and shut down nuclear plants. One of the options to replace coal plants is advanced water-cooled reactors, which have seen substantial improvements in design, such as passive safety. This is the capability of a reactor when you shut it down and cease the fission reactions that are generating heat, there continues to be decay of the fission products left over and there is residual heat created in the reactor core. If you don’t provide a mechanism for removing heat after it’s been shut down, the fuel can overheat. Active cooling safety systems have increased risks because they require an external source of power. As shown at Fukushima, there can be common mode failures of that emergency infrastructure, especially if preparation has not taken place.
24:35 Q6 – Nuclear Reactor Passive Safety
Bret Kugelmass: How does passive safety in nuclear reactors work?
Per Peterson: Experiments in the 1960’s showed that, if the fuel was not cooled, a lot of radioactive material could be mobilized. In water-cooled reactors that have zirconium tubes with uranium oxide pellets inside, overheating the tubes in a steam environment causes the zirconium to oxide, generating hydrogen. When the fuel overheats and melts, the fission products are cesium and iodine and take chemical forms in the steam environment, forming small particles that condense. This combination of high pressure and hydrogen mobilizes the fission products. The response was to increase a focus on the safety of infrastructure and high reliability emergency cooling systems. Back in the day, the best way to guarantee high reliability was to use redundant active components, such as pumps, which increases complexity and cost. Event trees allow calculations for a reliability number and adding redundancy to the system can increase that number. This creates a basis to assess the reliability and license the technology, but requires more power, increasing the size, power outlet, cost, and construction time. There are diseconomies of scale in nuclear reactor design. The new designs, like AP-1000, ESPWR, and NuScale, have a key change which was developing approaches to be able to quantify uncertainty in the performance of more complicated, passive safety systems. Integrated experiments were built to replicate the gravity-driven flows at a system scale and models were validated across a wide range of different transients. Oregon State University was the first university to use improved scaling methods and built integral effects test facilities that provided the data used in licensing of the AP-1000 and another test facility which produced the data used for NuScale. UC-Berkley has developed approaches to design and build similar integral effects test for molten salt cooled high temperature reactors in the Compact Integral Effects Test Facility. Virtually all of the advanced reactor designs implement passive safety and don’t require a supply of electrical power for cooling after a shutdown. The general approach to activating these shutdown cooling systems is to remove electrical power. Staff on the site must still have the ability to monitor the system and confirm it is in a safe state.
31:27 Q7 – Advanced Reactor Development
Bret Kugelmass: Is passive safety technologies being merged with current designs or are there fundamentally new designs that need to be brought forward?
Per Peterson: From the perspective of public policy, government has not been good at technology decisions. There are a number of market failures that make it challenging to develop new reactor technologies. NuScale recently submitted a $500 million license application, the cost of developing the application to request permission to build a reactor, shows that the industry has some challenges. NuScale has multiple reactor modules and developed a control room that has a total staff of six people. Their time motion studies show that they could respond to all contingencies, but the current Nuclear Regulatory Commission (NRC) regulations require that the control room have 48 operators in it. NuScale took risk in its application. When the NRC review the application and addresses the question, there is no way for NuScale to patent that regulatory decision and it is easy for competitors in small modular reactor (SMR) configurations to freeride. Freeriding is a market failure; first movers take on a lot of risk and can only capture a small portion of the rewards. Over the last decade, UC-Berkley has been working extensively looking at molten salt technologies for advanced fission reactors. Early work in molten salt came from aircraft nuclear propulsion program. Molten salt has high chemical stability and intrinsic low pressure because of high boiling temperatures, creating an ability to drive gas brayton cycle. Nuclear submarines were able to utilize existing technology, steam turbines and heat exchangers. When there was a strong interest in accelerating the development of commercial technology, the easiest thing at the time was to scale up water-cooled reactors, bringing disadvantages to light. Advanced high temperature fuels for helium-cooled reactors can be cooled by the same molten salts. The Department of Energy (DOE) has funded applied energy research, allowing UC-Berkley to establish a scientific and technical base for licensing molten salt reactors. A startup company founded by Per Peterson, Kairos Power, spun out and is pursuing molten salt technology.
39:22 Q8 – Parallels Between the Space and Nuclear Industries
Bret Kugelmass: What are key decisions and questions that Kairos Power needs to answer and how does it compare to the other three dozen technologies being developed?
Per Peterson: Some non-governmental organizations, like Third Way, Breakthrough Institute, and Nuclear Innovation Alliance, have been created to identify and solve generic policy and political problems that would benefit all of the advanced reactor developers. There has been a lot of support working with the Nuclear Regulatory Commission (NRC) to develop advanced reactor design criteria. The current Part 50 requirements were established for light water reactors and the design criteria is logical, but the way to accomplish that purpose may be different if it is a different reactor technology. The NRC has been working to update and develop specific design criteria for advanced reactors, which reduces risk for developers of advanced reactors. The Department of Energy (DOE) Gateway for Accelerated Innovation in Nuclear (GAIN) program gives a path for advanced nuclear developers to get access to federal and national lab resources. NASA had a similar program, Commercial Orbital Specialization Services, which gave birth to SpaceX. Nuclear technology is ripe for disruptive improvement. The question becomes how to innovate in a space in which, historically, very little innovation has taken place. For NASA’s program, the federal government was not telling startup companies how to do other technical work, nor were they picking which ones were the winner. This is very different than cost-plus procurement, which is the conventional way the federal government has developed new reactor technologies. Cost-plus procurement pays companies to do work and the longer it takes and the more you spend, the more you earn. In 2006, the United States knew the Space Shuttle was a failed technology and they had no alternative except to use Russian launch services. The conventional procurement program, Constellation, was spending billions of dollars and accomplished almost nothing. The strategic question for nuclear of what should be picked in terms of fuels, coolants, and technical approaches if you want to innovate more rapidly and develop new technologies which are disruptively better in terms of economic performance while having high intrinsic safety. Kaiser Power has its own thoughts about how to achieve that goal. The potential is very high, but there is also a potential for failure. SpaceX built three Falcon One rockets. The first, second, and third launches failed and SpaceX was out of money. They had enough spare parts to assemble one more rocket, which made it to orbit. Two weeks after their successful launch, NASA issued a multi-billion dollar contract to SpaceX for space station resupply missions.
49:02 Q9 – How to Support Nuclear Startups
Bret Kugelmass: Is there a lesson we can pull from the space industry to communicate to the nuclear startups, the public, and the government to make sure nuclear is given its fair shot forward as we continue to innovate?
Per Peterson: SpaceX could have never been successful if NASA had not had the capability to provide a procurement contract. SpaceX had to be able to perform. The Department of Energy (DOE) and the federal government have not figured out a similar way to do the same thing for advanced nuclear vendors. Absent those sorts of mechanisms, it could be very difficult for startup companies in the United States to demonstrate advanced reactors. The government needs to be an equal partner in terms of providing access to resources that U.S. startup companies can’t reasonably develop themselves, such as high enriched uranium. Terrapower, Bill Gates’ company, is developing advanced reactors and demonstrating them in China. The United States would be very proud of ourselves as a country if we are able to radically change nuclear energy as a technology at a faster pace and in a shorter timescale than most people believe is possible given the history of the industry.
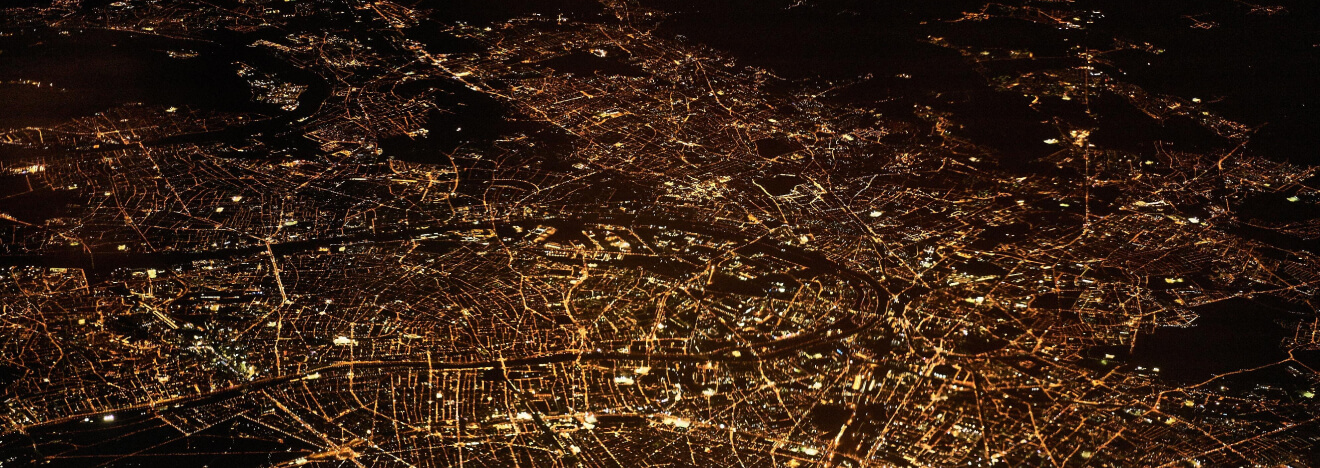