Ep 47: Michael Doser - Aegis Experiment (Antimatter), CERN
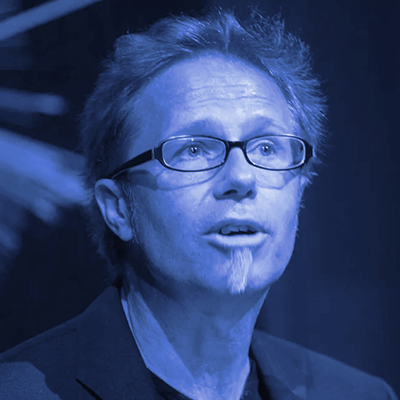
Show notes
Q1: How did you get into physics at CERN?
A1: Michael Doser studied Physics at ETH Zurich, then later received his PhD in Physics at the University of Zurich. He was sent to CERN to work on an experiment looking for mesons, which is looking for what kind of states quarks and antiquarks can form. Doser hoped to find states consisting of only force carriers, gluons. These glueballs were hypothesized to exist by annihilating protons with antiprotons. PET scans, which are used to look for high metabolic rates such as tumors or cell growth, are positrons annihilating inside the body. Doser started off at CERN as a high energy physicist working with antimatter, using it to study what holds nuclei together.
Q2: What brought you into the physics field to begin with?
A2: Michael Doser was initially Interested in molecular biology and computer science, but in 1978, there were not many high profile computer science labs and not many people working in molecular biology. Doser started programming at ETH with punch cards, at which point, physics became more interesting than programming. Doser ended up in particle physics as a high energy physicist. One realization in the physics world at the time was that atoms of antimatter could be formed. Hydrogen has a single proton and single electron, which is the simplest normal atom. Atoms of antimatter could be used explain some of the universe’s unknowns, potentially by comparing the differences between atoms of matter and antimatter. There is no antimatter left from the Big Bang, even though every time a particle is produced, an antiparticle is also produced.
Q3: What are some of these differences in characteristics that you’re trying to test between matter and antimatter?
A3: For a particle physicist such as Michael Doser, the universe is simply made up of particles, which can be identified by four characteristics: mass, charge, lifetime, and magnetic moment. Mass could be mass of an electron or an antielectron, but is the same value. The charge of an electron is negatively charged and an antielectron is positively charge. At this time, the charge is thought to be equal but opposite. Unstable particles, such as a neutron removed from a nucleus, have a probability to decay within a certain amount of time.
Q4: What is magnetic moment?
A4: Michael Doser’s studies center around the interaction of particles and its characteristics, such as magnetic moment. Particles are like a compass needle that follow a magnetic field. The strength of the coupling between the particle and the external magnetic field is the magnetic moment. They either interact with a strong interaction, a week interaction, or a gravitational interaction. The particle has a coupling between each interaction. Mass is considered to be the coupling between a particle and gravitational interaction. The difference between matter and antimatter, if it exists, is thought to be affected by external fields. An atom of anti-Hydrogren, which is neutral, will not have the same sensitivity to external fields as the charged particles of antielectron or an antielectron independently. The direct measurement of the gravitational interaction between matter and antimatter has never been taken.
Q5: What other projects do you work on at CERN?
A5: Michael Doser’s main project at CERN is studying the gravitational interactions between matter and antimatter. He is involved in other development projects needed to measure this interaction, such as laser cooling negatively charged molecules. These negatively charged systems are needed to make very cold anti-Hydrogen atoms to measure gravity. Gravity is a very weak force, so atoms have to be moving very slowly and studied over a long time in order to give the systems time to be affected by gravity.
Q6: What is antimatter doing at the center of the galaxy?
A6: Michael Doser Antimatter annihilates the antielectrons and positrons with the electrons, allowing us to see the antimatter from a great distance. An antiparticle can only annihilate once with another particle and there is no amplification effect. The International Space Station has an antimatter spectrometer is one example of many experiments currently ongoing in space. A “cloud of positrons” has been observed around the Earth, but the hypothesized closest location of antimatter is around Jupiter. If radioactive decay can be advanced technically, there is a potential to develop more positrons. There is a challenge of how to keep the produced antiparticles together due to their charge, unless they are combined to create anti-Hydrogen.
Q7: What medical applications exist for antimatter?
A7: Michael Doser sees one future use of antimatter in medical applications. Physiological processes in the body can be visualized via positron emission tomography (PET) scans. A radioisotope is inserted into a sugar which is inserted into the bloodstream. Cells that have a high need of energy are going to absorb the sugar. The radioisotope stays in that cell, decays at some point, and produces a positron. The positron annihilates with the closest electron and produces two photons, gamma rays, which can be detected outside the body. The two gamma rays emitted simultaneously allow the detectors to locate the source. The touchpad was invented at CERN in the 1970’s to control accelerators, which eventually was re-invented for the general population, but was not initially commercialized.
Q8: What is the application of a spallation source?
A8: Michael Doser’s work with anitmattter follows that of Carlo Rubbia in the late 1900’s. The spallation source was proposed by Carlo Rubbia, Nobel Prize winner for discover W and Z bosons by building an accelerator that collides protons and antiprotons. This “poor man’s collider” led to development of high energy physics. His next proposal was to use accelerators to drive the production of neutrons as protons are collided with a block in an accelerator, which generates lots of thermal neutrons. Neutrons create further neutrons through spallation and each fragmentation creates energy. Neutrons can also be used to fragment radioisotopes and transform them from long-lived radioisotopes into something shorter lived. By shortening the lifetime, the instantaneous release of energy is increased.
Q9: What else do you have to share about your experience with high energy physics?
A9: Michael Doser anticipates future applications of antimatter in medical treatment. Antimatter can also be used to treat tumors, which is traditionally treated with gamma rays. Gamma rays have a constant probability of being absorbed and deposits the same amount of energy where it stops. Enough energy has to be deposited inside the tumor to destroy the cells. This requires gamma ray therapy from multiple directions to attempt to minimize the impact on healthy cells. One alternative is proton therapy, or a carbon ion can be used in place of protons in proton therapy, but it is expensive to build the accelerators to strip the carbon atoms to obtain the carbon ion. Instead of protons, antiprotons could also be used for therapy. On their way in, they act as protons, slowing down gently and deposit all the remaining kinetic energy in the final 1 mm of movement. The antiprotons then annihilate and takes out several cells around it, maximizing the impact. Initial experiments show that antimatter therapy as the potential to be four times as effective as proton therapy, but more research is needed to duplicate real life conditions.
Q10: In normal proton therapy, how does a proton impact a cell?
A10: Michael Doser is currently studying the possibilities for antimatter in different applications. When a proton impacts a cell in proton therapy, it acts as a cannonball shot through sensitive, complex molecules causing single strand breaks in the DNA, and potentially double strand breaks. Cells can repair single strand breaks, but gives up once there are multiple double strand breaks and commits cell suicide. The development of antiproton production infrastructure is very expensive, considering the construction cost of the accelerator, building, and gantry used to move the beams in the therapy process. There are three types, sometimes called “flavors”, of standard neutrinos: electron neutrino, muon neutrino, and tau neutrino, plus their antimatter counterparts. Neutrinos oscillate from one “flavor” of neutrino to another, as do the antineutrinos. Fermilab is involved in an experiment call DUNE which will try to measure the oscillations for neutrinos and antineutrinos to identify if there are any differences in oscillations.
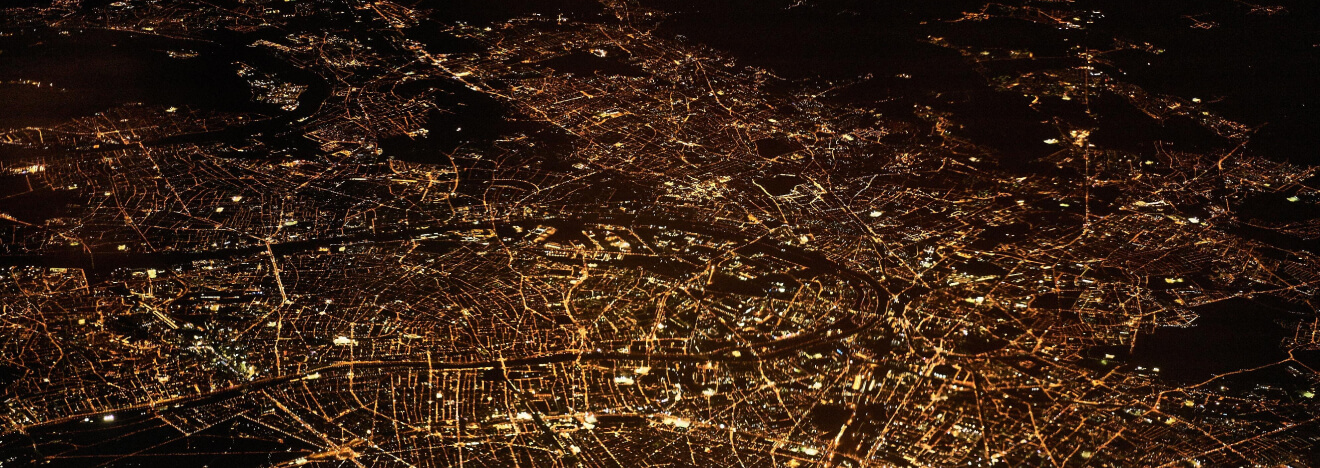