Ep 206: Koroush Shirvan - Assistant Professor, Massachusetts Institute of Technology

Show notes
Koroush enters the nuclear industry (0:13)
0:13-5:04 (Koroush explains how he entered the nuclear industry and what he learned as an intern at Southern Nuclear Headquarters.)
Q. How did you get into this space?
A. (0:18) Koroush Shirvan is a professor at the Massachusetts Institute of Technology (MIT). He teaches nuclear literacy to inform a wide audience about nuclear energy. His students include members of Congress, the media, think tanks and advocacy groups.
Koroush moved to Florida from Iran in 2001 when he was 14 years old because his parents wanted him to gain a strong education. Growing up, Koroush was highly interested in math, physics and engineering and ended up at the University of Florida because his aunt’s husband was a professor there and the head of Nuclear Engineering was also from Iran. Nuclear engineering also had many fellowships and financial support and created the opportunity for Koroush to intern at Southern Nuclear Headquarters. After the internship, Koroush was drawn to stay in the industry because of the interdisciplinary focus.
The internship at Southern Nuclear taught Koroush many things about the nuclear industry, particularly about reactor core designs. Roughly 40% of the fuel is removed from both Pressurised Water Reactors (PWRs) and Boiling Water Reactors (BWRs) when they are shut down every 18 and 24 months respectively. The fuel is placed in the spent fuel pools and new fuel is added. The arrangement of the fuel assemblies is important for performance, both for economic efficiency and to meet safety requirements.
Designing for optimization (5:05)
5:05-12:04 (Koroush describes the shift from designing for optimization to improving predictability. He explains what a secondary side is and why optimizing the design of this is important in today’s competitive nuclear landscape.)
Q. Is anyone looking at changing the geometric design of reactor cores?
A. (5:10) The core design focus changed in 2008 from designing for optimization to improving predictability. Castle, the Consorting for Advanced Simulation of Light Water Reactors based out of Oak Ridge National Laboratory, was created to enable more accurate predictions, which has been the focus of nuclear research and development (R&D) for the past 10 to 15 years. For new reactors, vessel size and old operation parameters no longer constrain design. For instance, the secondary side pressure no longer needs to be based on plant efficiency and safety limits.
Secondary side pressure is 6.5 to 7.5 megapascals, or about 1,000 psi, and half the pressure of a primary side. The saturation temperature in the primary side is 350°C, meaning the secondary side must have a lower pressure because the primary side is already below saturation. In newer plants, though, the secondary side pressure is actually higher than in old reactors, with efficiencies of 34 to 35% rather than 33% efficiencies in older plants. There is currently not much focus on optimizing the secondary side as the existing parameters are often adopted for new designs.
Koroush has worked with several vendors, learning that keeping designs the same instead of optimizing the secondary side often happens because existing designs have already been licensed by the regulator. Changing designs incur high regulatory costs involving both time and money. Simplifying designs and designing for inherent safety was a focus of the 1990s when competition was not as high as today. But because competition is stronger, optimizing the secondary side as much as possible should be a design focus.
Communicating nuclear safety (12:05)
12:05-19:11 (Koroush explains how the emphasis on safety arose in the nuclear industry. He also discusses the shift away from including safety in the nuclear communication strategy and his approach to teaching nuclear.)
Q. Did you track the history of the move to inherent or passive safety to understand where these notions came from and why we follow this pedagogy?
A. (12:10) Academic trends, such as the number of students in a particular major, are an indicator of how healthy an industry is. In the 1990s, the number of nuclear industry related students dropped because the public was not certain of the safety of the industry. Passive safety and probabilistic risk assessments stem from this rising public uncertainty.
No one has yet to create a perfect plan for communicating risk perception and changing people’s perceptions. It was once believed that communicating the safety of core damage frequency was a good marketing strategy. Like any other industry, there are groups advocating for different strategies, such as communicating nuclear in the same way as other industries. In Koroush’s class, students advocate for their particular state of mind. He leads the discussions in an unbiased way with the approach of teaching the subject rather than convincing students of a particular thing.
Academia versus industry (19:12)
19:12-28:03 (Koroush explains the interdisciplinary nature of the nuclear field and the differences between academia and industry. He also describes his PhD thesis and how the reactor design process has changed to focus on economics and safety.)
Q. What is the difference between studying nuclear as an undergraduate and a graduate student?
A. (19:15) Both of Koroush’s degrees focused on applications rather than just theory, but MIT gave him wider access to experts. Nuclear is highly interdisciplinary, requiring students to gain expertise in each engineering discipline as well as physics and policy. He believes he gained an even greater insight into nuclear engineering systems after his PhD when working in the field.
When learning something, teachings are often taken as truth and not questioned. In academia, much of the information is 10 to 15 years old and does not keep up with the continually upgraded equipment and procedures of existing nuclear plants. An example of a difference between what he learned in class and what he encountered in the industry comes from Koroush’s time as an intern. It is widely accepted that four new nuclear assemblies in a PWR are not placed together because it could create a hotspot, but Koroush has yet to see a physical justification for this rule. Additionally, the thought that designs should be as square as possible to reduce leakage is outdated because the decreased cost of uranium reduces the significance of possible leakage.
Koroush focused part of his PhD thesis on optimizing the geometry of the BWR design. The core design process used to flow in the following order: reactor physics, maximizing neutron economy, heat transfer, fuel analysis, safety analysis, risk analysis and economic analysis. Today’s process of objective function follows a different flow, focusing primarily on economics and meeting safety. Because prices have decreased over time, the core has become shorter with more pins closer together to create better economic performance. The size of the vessel in a BWR is critical because a short core means all other aspects are shorter, including the vessel, control plates, containment and spent fuel.
Startups and microreactors (28:04)
28:04-35:17 (Koroush states that both utilities and startups are exploring nuclear designs. He explains that startups that focus on microreactors are usually more successful because they require less employees and undergo an easier NRC licensing process.)
Q. Where do nuclear conversations take place?
A. (28:08) Conversations happen in many settings. For example, utilities have long term fuel purchasing agreements. Conversations must be both bottom-up and top-down because utility executives must be knowledgeable enough to renegotiate contracts as fuel prices drop over time. Designers focusing on creating core rods that are only 1 meter tall are found in utilities and in startups.
Koroush is familiar with the nuclear startup scene. The growing number of startups can be divided by the complexity of the problem that they’re focusing on and by the number of people in the startup. A large light water reactor may take millions of hours of work, so a small startup pursuing this type of reactor may not reach their goal. A startup focusing on micro reactors, on the other hand, could succeed with a fewer number of employees.
Microreactors also have an easier time than large reactors when it comes to demonstrating safety to the Nuclear Regulatory Commission (NRC). This is because the requirements to demonstrate safety rely on the reactor’s source term, which is the radionuclides that might escape from the reactor during a radioactive release and is directly proportional to the fission power generated by a reactor. The smaller the amount of power generated by a reactor, the less tricky NRC demonstrations become. Reactors that generate gigawatts, however, require a higher level of safety precautions to be demonstrated to the NRC. Microreactors may generate only 10 megawatts, meaning they undergo a less stringent NRC process due to the lower source term.
Calculating the initial source term involves the neutron cross section data and half life of radioactive isotopes. These are known, meaning good models can be created. Predicting mobility is more difficult. Low source terms, however, allow for other models, such as maximum credible release accidents, to be analyzed on conventional plume modeling to meet safety limits. Known engineering factors can be also used to estimate the dose profile.
Economic factors affecting new plant designs (35:18)
35:18-40:30 (Koroush describes the economic conclusions from his small reactor design research. He also explains why repurposing coal plants for new nuclear facilities have low economic incentives.)
Q. What are some of the conclusions that you have found about making reactors smaller and how does this affect economics?
A. (35:22) Koroush’s research resulted in a need for drastic design changes for utilities to remain competitive. Layout designs must be approached in a different way for small modular reactors to keep capital costs low and to overcome the economies of scale that large plants benefit from.
Repurposing coal plants to become nuclear facilities has been looked into, but economics explain why this does not occur. The turbine island is low cost to begin with, but introducing it to an existing coal plant would require reconfiguration costs. Additionally, the connection between the turbine island and the control room must be updated, which introduces a new cost. Overall, there is not much economic incentive to repurpose a coal plant unless the site underwent a high cost excavation to begin with.
Repurposing reactor fuel (40:31)
40:31-51:04 (Koroush discusses the various reasons behind reusing fuel. He also explains the conversion ratio, the factors that limit the number of fuel cycles and why mixing fuel pellets with coolant is not a practical solution.)
Q. How do you think about the different fuel cycles?
A. (40:34) Koroush has only worked with one reactor design as a long term project that could burn repurposed reactor fuel. The Reduced Boiling Water Reactor designed by Hitachi boiled water to increase the flight time of neutrons, improving the efficiency of disposing plutonium and burning nuclear waste. Decisions to reuse fuel are based on cost, so reprocessing fuel does not make economic sense when uranium is cheap. Other factors, such as not wanting to store plutonium, will influence the decision to repurpose and burn used fuel.
Light water reactors are proliferation resistant to a certain extent. The conversion ratio of a light water reactor is 0.3 to 0.4, meaning they burn fuel efficiently. The conversion ratio is the ratio of the amount of fissile material that a reactor begins with versus the amount it ends with. The breeding ratio and conversion ratio are essentially the same. If the conversion rate is 1, the amount of fissile material is the same at the end. This is good from a fuel economic standpoint, but means fuel may need to be reprocessed, requiring additional cost and energy.
There are many limitations that restrict the number of fuel cycles. The burnup, or the amount of energy extracted per kilogram, must be increased. This is limited by the capability of uranium dioxide to hold onto its structure. It is also limited by the metallic cladding material’s structure to hold during accident scenarios. Continuing to burn low fissile inventories requires other assemblies to generate more power, creating more hotspots. This is further limited by the thermal margin, which is in turn limited by the fuel properties.
Mixing fuel pellets with coolant presents potential problems. If the cladding breaches during an accident, water would pick up the pellet grains and contaminate the entire system. Theoretically, pump seals could also erode, leaking radioactive fuel.
Accelerating innovation (52:05)
52:05-59:17 (Koroush discusses what needs to happen to accelerate innovation and secure the future of the nuclear industry.)
Q. How do you think innovation can be accelerated in the sector?
A. (52:11) Koroush emphasises the need for an integrated approach with any new technology. Incorporating both the economic and safety viewpoints avoids unnecessary steps and removes circular cycles. Koroush saw the nuclear renaissance of the 2000s, creating a profitable era for utilities and R&D investment. Koroush now asks why those funds were not used to secure the future of nuclear. He sees the need for a coordinated effort between government and industry to support R&D. Additionally, Koroush believes that if the public perception problem is fixed, the critical next step is attracting the best engineers to the sector. This will bring about investment in technology and strong management skills to best execute projects. This will lead to reactors being built on time and on budget.
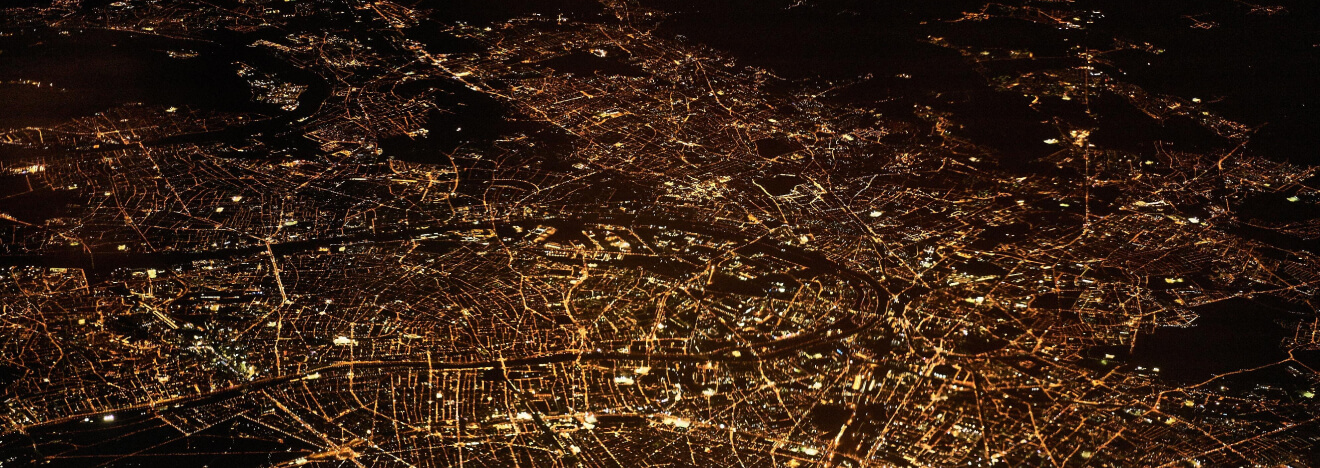