Ep 36: John Stevens - Reactor Materials, Argonne National Lab
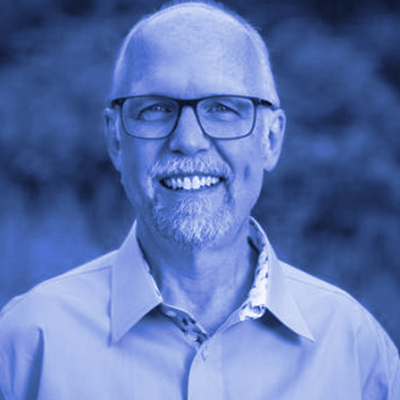
Show notes
"Q1: How did you get involved in the nuclear industry?
A1: John Stevens attended high school in the Washington, D.C. area and got a summer job working at the radiocarbon dating laboratory for the Geological Survey. Stevens pursued his undergraduate and graduate degrees at Purdue University with a focus on nuclear engineering. His first co-op was with Westinghouse Nuclear doing reload fuel design. In one reactor cycle, only about one-third of the fuel is used up enough to be replaced. Eventually, enough Uranium-235 has been used up and the fuel must be discharged.
Q2: Tell us about your work with nuclear reactor fuels.
A2: John Stevens considers himself an environmentalist, focused on digging up as little as possible and burying as little as possible in order to get the energy needed. The first reactors to produce electricity in the U.S., EBR-1 and EBR-2, were fast reactors using liquid metal coolants. The core reload process, moving new fuel in and old fuel out of the system, can save a significant amount of money if completed efficiently. John Stevens also spent some time developing and improving simulations at Studtsvik of America, a software branch that utilized original simulators from Studtsvik nuclear site in Sweden.
Q3: What is the process of erosion that takes place on nuclear fuel rods?
A3: John Stevens observes that, within light water reactor systems (LWR’s), there will be a reactivity limit to where the U-235 is consumed and the Plutonium being made in that system. Odd-numbered Plutonium isotopes are good for producing power, and the the even-numbered isotopes are good at absorbing. LWR’s build up more actinides that will absorb neutrons than those that will produce good energy. Taking useful material from the used fuel in the LWR’s and putting it into fast reactors gets the energy value out and fission those elements to turn them into shorter-lived waste products. Slow neutrons, when they hit actinides, are absorbed more often than they cause fission. Fast neutrons, won’t be absorbed when they hit actinides, and may bounce off, but there is a probability that they will induce fission. A combination of speed, relative speed, angle, and temperature, among other factors, can affect this probability.
Q4: You helped design this software that mapped out where fuel rods are moving and the effect on their longevity. Is it a database that keeps track of where each fuel rod has been through the life of the reactor?
A4: While John Stevens worked at Studtsvik of America, he helped design a software to map out where fuel rods are moving and the effect on their longevity. The Studtsvik model of software had an individual pin level of the fuel level, but in a light water reactor, hundreds of pins make up a bundle and hundreds of bundles make up a reactor. The software had to be simplified to look at the core, rather than at the pin, and became the software called Simulate. Later add-ons included a database of each pin, a point-and-click allowing an operator to move fuels and see the effects, and then an automated version of this optimization software. Once computers got fast enough, operators could predict the effects of changes in the reactor. The software could be used to see if a reactor could effectively use fuel from a different vendor.
Q5: What is the complexity of the nuclear component of a power plants versus the whole system itself?
A5: John Stevens saw the U.S. embrace innovation in the 1970’s with the light water-cooled reactors, which were all designed and created differently. The regulator has to look at the full system, creating a need for a level of complexity in the analysis of things that could go wrong and losing the economies of scale by treating each reactor uniquely. France, instead, deployed a small number of different system designs for their plants. The U.S. innovation culture allowed technology to evolve quickly, but by provisioning a large number of variants, an unintended consequence was a complexity in the system and a difficult regulatory system. Small modular reactors (SMR’s) might have a lot more similarity in manufacturing to improve this issue.
Q6: What kind of work did you do with research reactors?
A6: After John Stevens’ 16 years at Studtsvik, Stevens took an opportunity to consult on a reactor conversion of a research reactor in Portugal. Part of Eisenhower’s Atoms for Peace put forward an initiative that the U.S. would help countries with nuclear science who forego nuclear weapons programs. Hundreds of these low-enriched fuel reactors were built around the world. There are scientific reasons that U-235 at a high concentration in the fuel is better for scientific purpose, compared to low-enriched that has a lot of U-238. The number of neutrons inducing fission, rather than being absorbed in the fuel, and come from the same species with the same initial energy. A compact core can have a stable neutron spectrum, which is valuable for normalized results in research and the neutrons can leave the fuel and be used for other things, such as producing industrial quantities of doped silicon.
Q7: When the neutron smacks an atom, it displaces the lattice structure, causing it to be brittle and weaker. How does self-healing happen in certain alloys?
A7: John Stevens sees that some materials are much better and being able to recover their structure, while some will develop faults. Studies were completed in these research reactors to see how different materials reacted in a nuclear environment. Fission research reactors are being used to help understand the materials that will be used in fusion devices, which are highly radioactive. Research reactors can also be used for isotope production, for which we need the reactors to have the neutrons that get absorbed by one element and either the next product formed, or a daughter product, is useful. One example is the MUR Reactor in Missouri, which figured out how to irradiate exotic materials to produce a radioactive isotope with a high energy and very short range that can be implanted in a grain of rice and used to treat prostate cancer.
Q8: When Eisenhower gave his Atoms for Peace speech, did anyone know at the time all the possible applications?
A8: John Stevens believes that, at that time of Eisenhower’s Atoms for Peace speech, scientists did not know the full range of isotope applications, but had figured out that there were going to be useful isotopes. Smoke detectors have Americium-241, which is a reactor-produced isotope which produces Alpha particles at a steady rate and becomes blocked by smoke particles in event of a fire. Another example is Technetium-99, which is a radioactive contrast used for medical stress test procedures.
Q9: Tell me about the program you currently work on, which converts high-level enrichment reactors into low-enrichment reactors.
A9: John Stevens goal in his current position is to maintain the scientific effectiveness of research reactors, but eliminate the risk that the fuel product in the reactor could be diverted to be used for weapons use. In the 1990’s, China exported Miniature Neutron Source Reactors to places like Ghana, Nigeria, Pakistan, Iran, and Syria. Stevens’ team and China are working together to convert these reactors. Fuel alloys and altered fabrication techniques can alter the fuel density, allowing reactors to meet their mission with a new fuel that is low-enriched Uranium.
Q10: Was there a discussion of evaluating whether it would have been easier to provide a new research reactor instead of converting reactors from high-enriched to low-enriched?
A10: John Stevens currently works to convert high-enriched Uranium research reactors into low-enriched reactors, which was determined more cost-effective compared to provide a new fleet of research reactors. This program at Argonne National Labs has converted approximately 70 reactors since the program’s start in 1979, and 28 reactors chose to shut down instead of be converted due to lack of use. Knowledge is taken from these conversions into the design for new test reactors. Fuel qualification takes approximately a decade, and about 50 more reactors are in the process of conversion. Pulling together the safety and security of the reactors to eliminate proliferation risk will create a sustainable nuclear reactor. "
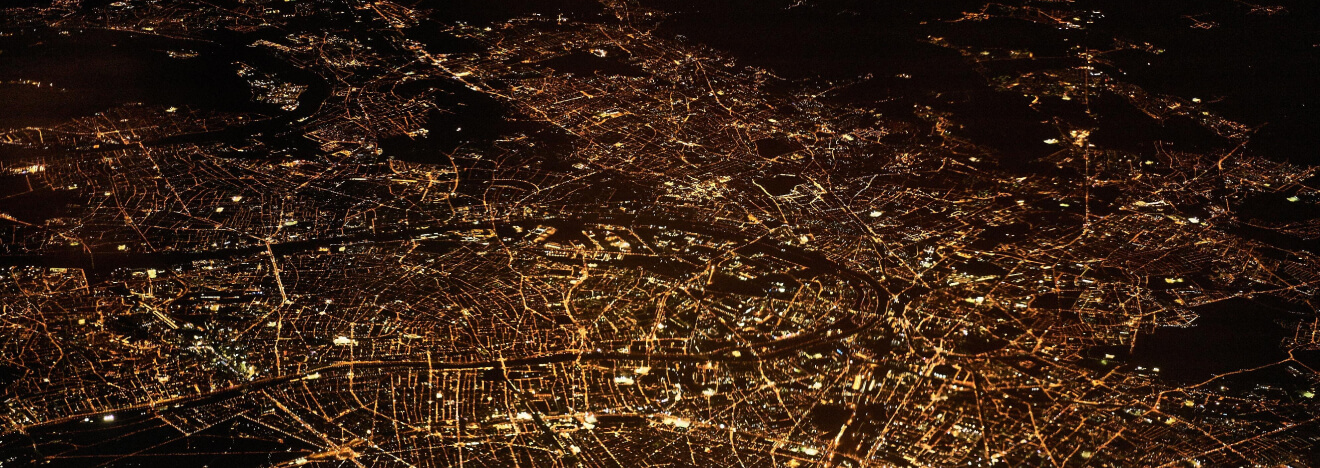