Ep 70: Ian Scott - Co-Founder, CTO, Moltex Energy
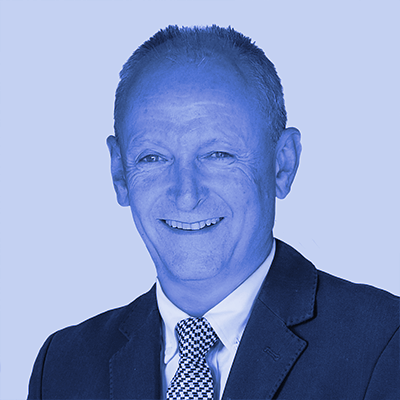
Show notes
Q1 - Entrance into Nuclear
Bret Kugelmass: How did you start out in the pharmaceutical and biology industry?
Ian Scott: Ian Scott’s first career was with Unilever, where he became one of four chief scientists for the company. Scott left in 2003 because he wanted to go back to the lab and do real experiments instead of managing. This took Scott into the biotech field and to a company called Synergy Biosystems, which looked at high order synergies among biologically active ingredients. After ten years, Scott retired shortly before seeing an article saying that EDF Energy in the UK were going to be paid nine and a half pence per kilowatt hour from Hinkley Point C nuclear power station. Scott became interested in why nuclear had gotten ludicrously expensive. Scott’s intention at university in Cambridge was to study particle physics because it looked fascinating to him. During his first year, scientists basically solved particle physics with the standard model. There were gaps, including Higgs Boson, but Scott saw that the next forty years would just be filling in the gaps.
Q2 - The Economics of Molten Salt Reactors
Bret Kugelmass: What did you see in nuclear in terms of the electricity problem?
Ian Scott: Ian Scott saw nuclear energy as an economic problem for how it would fit into the electricity space. Nuclear fission itself is simple, but the challenge is how to do it economically. In the early 1970’s, the price per kilowatt of building a nuclear power station was cheaper than the price of building a coal-fired power station. Nuclear made economic sense, until Chernobyl, Fukushima, and Three Mile Island happened. The early, cheap reactors were great until they weren’t. The industry fixed the problems, but at a high cost and priced nuclear out of the market. Pressurized water reactors are government vanity projects and are not market driven. Scott reviewed every concept for nuclear reactor that had been published and saw reasons why every single one would never crack the economic problem, such as a major engineering issue that would pile cost into the design. The one thing that caught Scott’s eye was molten salt fuel. Uranium can be used just as a metal, or it can be turned into a compound, such as uranium oxide, to form a ceramic, which is used in most light water reactors. Uranium oxide holds the fission products in the form of a very high pressure gas, which is dangerous if released. Molten salt sticks to uranium and turns to a different chemical, chloride or fluoride. These are salts and at the temperature a reactor operates are molten and the fission products, cesium and iodine, are no longer gases. If the containment of the molten salt fuel is breached and a hole is knocked in the concrete containment of the reactor pressure vessel, there will not get a serious reading of radioactivity at the boundary of the site. There is an intrinsic safety to molten salt fuel. One of these reactors was made operational at Oak Ridge National Lab in the U.S., the Molten Salt Breeder Reactor. Molten salt fuel must be pumped around and reactors create extremely radioactive spent fuel, which is dangerous if there is a leak. The key insight leading to Moltex Energy was that the whole paradigm of having to pump the molten salt around was false, but the salt fuel can instead be put in tubes in fuel assemblies in the reactor core and removed when they are burned up.
Q3 - Molten Salt Reactor Coolant Systems
Bret Kugelmass: What kind of coolant is used in a molten salt reactor?
Ian Scott: Molten salt coolant, without any fission products, is used in molten salt reactors. The metal tubes containing molten salt with fission products are placed in molten salt coolant. Ordinary stainless steel swells in radioactive environments, so ferritic steel is used instead, since it has good properties in a radiation field. To get the heat out of the system and create electricity, coolant is circulated throughout the core, passes it to a second molten salt coolant loop, and then goes to a third molten salt loop, which has solar salt. The third loop is used to get it to large tanks, which double as a storage system to allow for grid flexibility. Battery storage is still too expensive and it has significant carbon and waste footprints. Electricity cannot be stored at a large scale economically, but heat can be stored in solar salt. Being able to dispatch nuclear power when the renewables are off and the demand is high makes a big difference in economics and capital costs. A 300 MW nuclear reactor with a large storage system could be paired with a 900 MW steam turbine power station. The total plant cost per kilowatt electricity, including storage and turbines, goes down a lot because the cost of the nuclear plant is being divided among three times as much power as its producing. Steam systems have to be inside a containment because they are radioactive and highly pressurized. This containment is usually a meter thick, heavily reinforced nuclear concrete structure, which costs a fortune, and contains the steam generators, heat exchangers, and depressurizers. By taking the steam system away from the reactor, it is not a nuclear regulated phenomena.
Q4 - Molten Salt Reactor Life Cycle
Bret Kugelmass: Why don’t pressure water reactors add another heat transfer loop of some sort of salt and move everything out of the containment dome?
Ian Scott: Pressure water reactors run at low temperatures, with an output temperature of about 300 degrees centigrade. The temperature must be around 600 degrees for the heat storage to work. Ian Scott’s molten salt system is different than other molten salt systems because of the differences in pumping. For salts to be pumped, a reaction chamber is needed and must last the life of the reactor. Terrestrial Energy’s concept is to throw away the whole reactor system every seven years, but there is an issue of whether you can pay for building, running, and decommissioning it based on seven years of output. Scott’s reactor is designed to last sixty years, since the bit that holds the fuel is in fuel assemblies and come out the reactor every five or ten years, just like conventional reactor fuel. Because of the intrinsic safety of molten salt reactors, there is no pressure in the core. Scott’s design uses chloride, because his reactors are fast spectrum reactors which do not have a moderator. This requires a higher amount of fissile material in the core, but also burns up the long-lived actinide which accumulates in the waste and thermal reactors. This platform technology allows for both fast and thermal reactors the use of chloride and fluoride fuels. Ian Scott uses chloride fuels because it can be made very strongly reducing to avoid corroding metals. Chemistry must be adjusted to avoid uranium metal and chromium chloride, but instead to have equilibrium of that reaction of chromium metal and uranium chloride. This is harder to do with fluoride.
Q5 - Nuclear Fuel Qualification
Bret Kugelmass: Have molten salt fuel experiments been completely modeled out?
Ian Scott: Qualification of the fuel is one of the hardest parts of developing a new reactor. For a uranium oxide fuel, it needs to be put into a reactor to see how the pellets behave when they’re being fissioned. This cylindrical pellets have lots of cracks and swell until they touch the wall of the metal cladding; if the fuel is not right, it can rupture the walls. Molten salt fuel is a liquid and has no pressures. This type of metal can be tested in two ways: if it causes corrosion of metal, and what happens to the liquid as it fissions. The things that make qualification of fuel hard don’t apply to molten salt fuel. Nuclear regulation is entirely a national responsibility and different regulators operate very different systems. The U.S. regulators went down a route for regulation in which they wrote out rules, and if the rules are passed, the reactor is safe and it will get approved. The problem is that the rules were written for pressurized water reactors and to approve a different reactor, one must produce a new set of rules. The nuclear regulators in U.K. and Canada operate similarly, which have no rules, but a case must be assembled to prove your reactor is safe. This system is better for new technology and those that have intrinsic safety principles. One of the problems with nuclear is that everyone thinks it’s the government’s job to develop new nuclear technology. Its roots and culture were in the military complex.
Q6 - Controlling Nuclear Construction Cost
Bret Kugelmass: How do you know how much your plant is going to cost?
Ian Scott: In mega project territory engineering, times two cost overrun is almost through for any sector, including nuclear reactors, bridges, and tunnels. These projects are so big and complex that they just tend to go wrong. If the reactor can be smaller, there is more predictability in terms of cost and reduces the construction period. Ian Scott’s molten salt reactor has a construction period of three and a half years. The industry as a high experience rate and competency for building turbine systems, which is approximately 60% of the construction cost. Coal-fired or oil-fired gas plants could be modified by swapping out the coal furnace with a nuclear reactor and turning it into a nuclear power station. In order to do this practically, the steam island must be functionally separated from nuclear. If the reactor shuts down, steam turbines cannot be used to get rid of decay heat. Once you make a small claim on the turbine system to keep the reactor safe, it becomes nuclear. Molten salt storage tanks allow steam turbines to be separated from the reactor. China is building a lot of coal-fired power stations because they don’t want to tank the economy, even as they want to move away from nuclear. They want to take sunk capital costs and go carbon free with a nuclear retrofit. If nuclear can be cheap and utilize stored energy, Ian Scott sees no reason by the world is anything other than wind, solar, and nuclear power. This gives a complete, robust energy system with zero fossil fuel burning. This is a future in which consumers spend less on their electricity than they do now; cheap electricity brings higher quality of living.
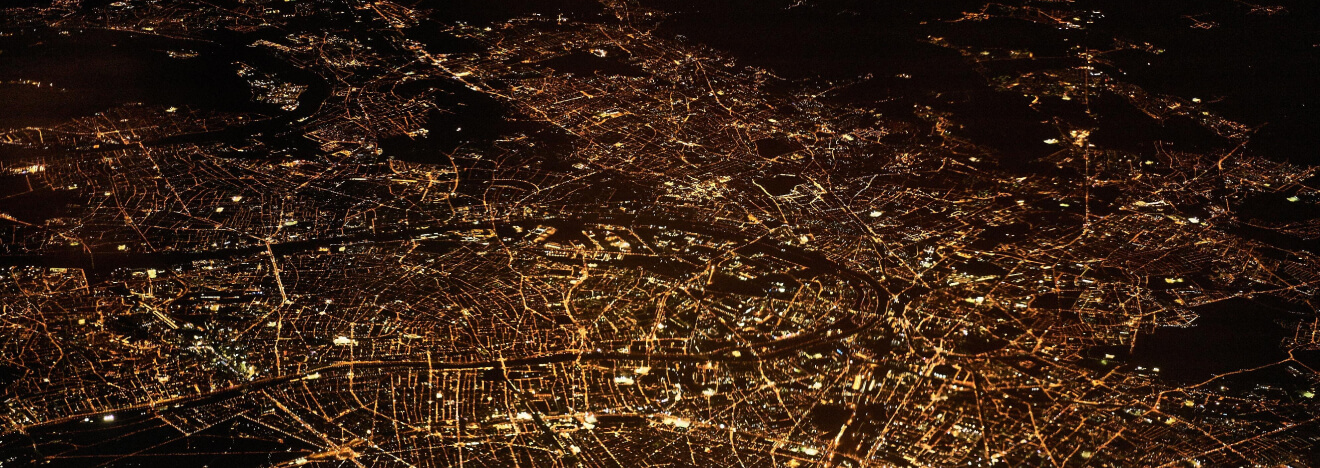