Ep 212: Doug Witherspoon - CEO & Chief Scientist , HyperJet Fusion Corporation
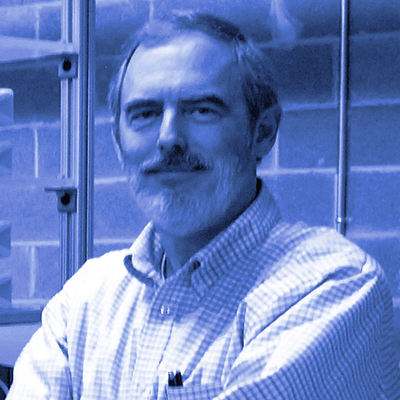
Show notes
A career in fusion technology (0:43)
0:43-8:56 (Doug describes the specific fusion work of HyperJet Fusion. He also explains how he got into fusion technology.)
Q. Your specific style of fusion is pulsed fusion?
A. Doug Witherspoon is the CEO & chief scientist of HyperJet Fusion. He studies pulsed fusion, known as plasma jet magneto inertial fusion (PJMIF).
After graduate school, Doug worked for a small company researching impact fusion. Impact fusion is when high velocity projectiles impact a target to create fusion energy. The target Doug used had deuterium tritium fuel inside a metallic cavity and the physical projectile was solid metal and plastic. The projectile fuses when it hits the target, creating fusion energy. The walls of the chamber absorb this energy, which is the kinetic energy of the neutrons. This energy is then transferred to the heat transformer, which makes steam and drives an electric generator. Doug’s work discovered that the target was hard to hit at high velocity, about 10 to 20 kilometers. The company then decided to pivot towards developing hypervelocity guns for the Department of Defense. General Dynamics Land Systems purchased the company to create a new generation of rail guns. A few years later, Doug left to start UTRON to develop a combustion light gas gun for the Navy. During this time, Doug also began exploring pulse plasma discharges for materials applications such as producing metallic powders for thermal spray and dynamic compaction, which is when metallic powder is compacted into a part using combustion light gas gun technology. In 2003, Doug left UTRON to pursue fusion technology.
HyperV Technologies (8:57)
8:57-15:37 (Doug explains why he pursues fusion technology and the current focus of HyperV Technologies.)
Q. What drives you to fusion and why is it important to you?
A. Doug believes fusion is a good energy source and focused his graduate school thesis on the topic. After Doug left UTRON, he started HyperV Technologies. Doug wanted to focus on fusion research in the realm of hypervelocity. Doug found Frances Theo’s ideas about plasma jet magneto inertial fusion (PJMIF) interesting, especially his ideas about developing the plasma gun for PJMIF. This gun is used to accelerate the gaseous plasma of xenon or argon to 50 to 100 kilometers per second. The gas is ignited and the magnetic force from a current accelerates the plasma along the plasma tube, driving the implosion. The j cross b force describes the directions of the current, the magnetic field and the force. The force is the direction of acceleration and the current and magnetic field are perpendicular to the force, but are in different directions to one another. This force creates a liner, which is a shell that is rapidly imploding. The shell is kept spherical by beginning as smooth as possible. Doug’s current experiment involves 36 guns, but between 300 and 600 guns will be needed for a fusion reactor. The current experiment involves a 9 foot diameter vacuum chamber with 60 gun ports and Doug hopes the following year will bring additional funding for more guns.
PJMIF explained (15:38)
15:38-25:47 (Doug explains details of PJMIF, including the physics and components of the system.)
Q. How do you keep the fuel in the center of the vacuum bubble?
A. It’s inertial. A subset of the guns are uniformly distributed and fire a mix of plasma-form deuterium and tritium before the other guns fire. This creates a preliner, which collapses on the center. This then causes the center to collapse into a small ball of plasma that lasts only long enough for the real liner to collapse onto it. This process happens on the microsecond scale to quickly capture the plasma ball before it expands. Only a few milligrams of fuel is inside this plasma ball. The percentage of fused material depends on final conditions, but a substantial fraction of the deuterium tritium fuel will fuse.
The amount of energy released in a pulse depends on the reactor design. If the guns were firing at the rate of one shot per second, the average energy output would be between 50 megawatts and one gigawatt of thermal energy, which would then be converted into electricity. Most of this energy is in neutrons and alpha particles. The alpha particles could be converted into electricity directly if a magnetic field was in place, but this is not easy to do as most of the energy is produced as neutrons. The kinetic energy must be absorbed using a thermal blanket. This blanket sits outside of the vacuum. This thermal blanket is hooked up to a steam cycle where molten salt absorbs the energy, which is then cycled through a heat exchanger. This converts water into steam to drive a generator.
Because the guns fire in pulses, there is no helium ash problem. The chamber is completely evacuated between shots. This evacuation therefore limits how fast pulse cycles can occur. Despite this, there is not much to evacuate because only the deuterium tritium fuel and the liner plasma, which are all gaseous, enter the vacuum. For PJMIF to work, the vacuum only needs microtour because only the residual argon atoms could get in the way. The walls of the vacuum are cleaned and any left over water would disappear quickly after firing begins.
Three development challenges to overcome (25:48)
25:48-35:51 (Doug explains three development challenges that must first be overcome before a fusion reactor can be brought to market.)
Q. What are some of the challenges in bringing this to market?
A. The main complication is creating a liner that is sufficiently smooth to avoid instabilities. A low density gas that is brought together with a high density gas can form surface ripples, but merging the gases fast enough can avoid this as long as the liner is smooth enough to begin with. This is still in the experimentation and modeling phase and still needs to be resolved.
The second issue is how to form the target, which must be magnetized with an embedded magnetic field that increases in strength as it is compressed. The process must be fast and can not lose energy to maintain heat. Understanding this magnetic property must still be researched. One area of focus is developing a traveling wave using lasers to drive electrons within the plasma, creating a current and the magnetic field. Doug is planning to place a loop of current at the gun’s muzzle with the hopes that the magnetic field will reach the target as it travels with the plasma jet.
The third challenge is the development of the guns. Each gun must accelerate at least 20 milligrams of plasma at about 100 kilometers per second using a compact jet once per second. This creates issues of cooling and achieving the required parameters. The repetition rate will be the most difficult to achieve. The guns would require a different coolant loop to the reactor and it is currently unclear what coolant fluid would be used. The guns would not want to exceed a few hundred degrees celsius because gun seals are usually rubber and must be kept under a certain temperature.
Accelerating progress requires additional funding (35:52)
35:52-41:10 (Doug discusses how funding is required to move fusion technologies forward and accelerate progress.)
Q. What will move this technology forwards?
A. Doug’s work is funded by ARPA-E’s Alpha Project. There is currently work to plan a following program that could fund Doug to further develop the target guns. Doug does not expect to produce neutrons in the next program because he does not foresee the project reaching the necessary parameters. While they would love to see neutrons being produced at this stage, the facility can not handle too many neutrons because it presents a safety issue. Rather, the next step is focusing on getting the liner on the target.
Even with large sums of money, the challenges will not be overcome within five years. This is because much of the work must be completed in series. The process can be sped up and Doug predicts that progress can be achieved within seven or eight years. Funding is the primary issue in achieving this goal. More people are needed to code simulations as well as to develop gas valve, igniter, capacitor and switch technologies. Much of this work could be done in parallel to the plasma implosion process development. Doug believes there are talented scientists within the community, but the financial support is currently lacking.
Advantages and disadvantages of PJMIF (41:11)
41:11-46:28 (Doug explains the advantages and disadvantages of HyperV Technologies’ system compared to other fusion approaches. He also states his hope for the next 15 years.)
Q. How does this technology hold up against the other approaches to fusion?
A. There are both advantages and disadvantages. Magneto inertial fusion has the potential to reach the fusion reactor stage sooner and cheaper than the Tokamaks, which is costly in terms of time and money to build. Doug’s approach allows for faster research and development, meaning the point of break even can be reached sooner. Each approach has similar physics-based challenges. Doug’s primary focus is an engineering challenge to create a system that performs as wanted. Doug expects a reactor with this technology to be not much smaller than 50 megawatts and could fit into about the size of an area slightly bigger than a basketball court. The system could be about 10 meters in diameter, but the support systems and structure add to the size. Fifteen years from now, Doug believes fusion will reach the break-even point.
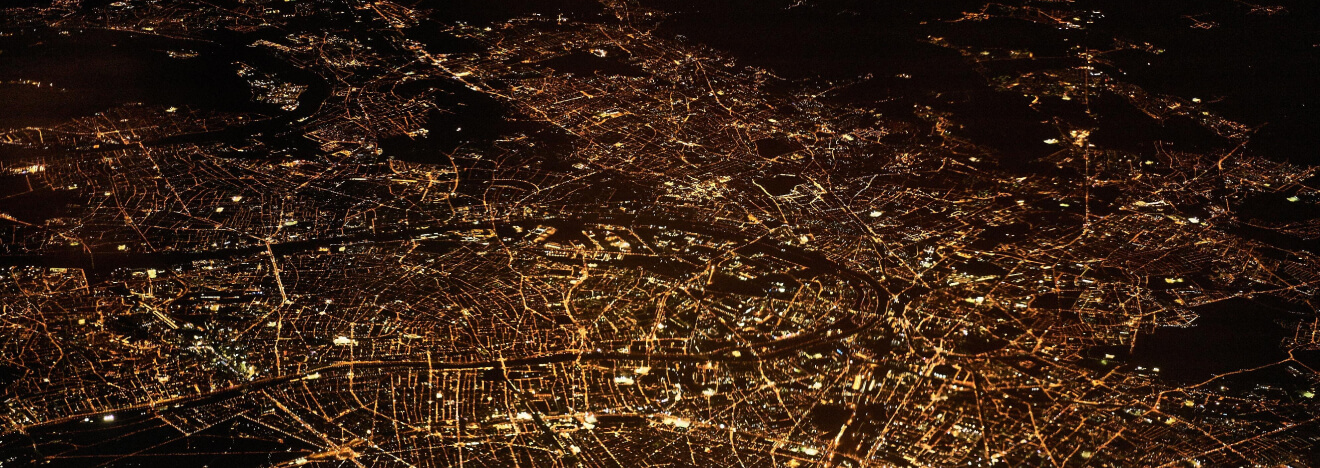