Ep 16: Don Wolf - Chief Executive Officer, Advanced Reactor Concepts

Show notes
0:00 – Advanced Reactor Concepts
Bret Kugelmass: Tell me about your nuclear technology.
Don Wolf: Don Wolf’s neighbor, a consultant to Sandia National Laboratories, was approached by SNL about a need for a reactor that could be operated very simply to be deployed in Libya. SNL envisioned it with a long refueling cycle, be located underground, and used with sodium coolant. This reactor was going to be delivered by a full 20 years of fuel and taken away at the end of the 20 years. When the cartridge was removed, scientists would use an electrorefining process to separate the heavy metals from the products of fission, producing one electrode with heavy metals and one electrode with fission products. Fission products are removed and the rest of the material can be placed into new fuel. These fission products have a half-life in the hundreds of years, as opposed to other elements that come out that having half-lives in the hundreds of thousands of years.
0:00 – Liquid Sodium Pool-Type Reactors
Bret Kugelmass: How is your nuclear technology configured?
Don Wolf: Advanced Reactor Concepts (ARC) conceived a reactor that had 100 MW, a size that worked on many different grids, which is a liquid sodium-filled tank sized 25 feet in diameter and 45 feet in height. Sodium is a solid metal at room temperature, and melts at 98 degrees Centigrade, staying in the liquid state up to 900 degrees Centigrade. The temperature operating range in ARC’s reactor is 300-500 degrees Centigrade and operates at atmospheric pressure. The tank is not a 12” thick forged steel, which is difficult and expensive to fabricate, but instead is a 1” thick stainless steel tank that can be manufactured almost anywhere. The vessel is a pool-type design and all piping is inside the tank. Sodium reacts energetically with moisture and air. On top of the tank, there is a layer of argon to protect any air from entering the tank. Argon is heavier than air, meaning air doesn’t go through it and it stays on top of the sodium. A few years after Three Mile Island, an experiment took place to test the prototype reactor at different temperatures. In addition to the pool-type design and sodium coolant instead of water, the reactor used metallic uranium fuel as opposed to uranium oxide. When metallic uranium fuel gets hot, it expands and has very good heat transfer characteristics, but the fuel rods kept cracking. Sodium and uranium metal are chemically non-reactive, compared to problems that come with uranium oxide leaks into water. To solve the expansion problem, liquid sodium was placed in the rods with the uranium. Inside the uranium metal where fissions take place, the heat generated transfers efficiently from the center to the outside, where it contacts sodium metal, goes through it with very little loss of temperature, through the stainless steel rod, and into the liquid sodium. Having a low centerline temperature is key to the inherent safety of the reactor. All accident sequences are accompanied by an increase in temperature and heat; getting this heat out prevents a meltdown. The fuel pins start to get hot, expand, and the molecular dissonances between the atoms start to increase. When there is a fission, some of the products escape and are not captured by a uranium ion, causing neutrons to leak. This is known as negative reactivity due to neutron leakage. When the Doppler Effect is strong and the temperature of the fuel starts to drop as it is cooled, it becomes more attractive to neutrons and grabs them more readily. This negative reactivity temperature dependent and does not happen in a water-cooled reactor. As the heat comes on, the neutron leakage kicks in, temperature rises and levels, and can be brought down to zero average power or be turned off. The original demonstration for this reactor was completed in Idaho shortly after Three Mile Island and right before Chernobyl at the Experimental Breeder Reactor (EBR-2). As power was cut off, the coolant flow slowed down. Temperature started to rise from 500 to 650 degrees Centigrade and leveled out as the neutron leakage effect and negative reactivity combined to arrest the increase and offset the temperature rise. Shortly after, it dropped back to 500 degrees. At Fukushima, the reactor scrammed, but they ran out of electricity and couldn’t get rid of the decay heat. The demonstration also duplicated Three Mile Island incident, a loss of heat sink, by turning off the turbines that extracted the heat. The sodium flowed over the fuel pins, but there was no heat being extracted. Once temperature rose, the neutron leakage effect took place and arrested the temperature rise. This reactor also automatically responds to changes in electricity demand, called load following, in a way that doesn’t require the adjustment of control rods. The grid starts extracting more heat from the sodium, and when the sodium comes back cooler, the molecular dissonances decrease, creating more reactivity. This reactor ran after the safety demonstration until 1993.
0:00 – The Return of the Experimental Breeder Reactor-2 Technology
Bret Kugelmass: How did you get involved with this reactor?
Don Wolf: Sandia National Laboratories wanted to bring the reactor back and Don Wolf recruited three individuals that had done the original EBR-2 reactor and one man from Argonne National Laboratory in Chicago. This group later teamed up with General Electric, who had taken the same EBR-2 technology and developed it into a reactor that came very close to being licensed by the Nuclear Regulatory Commission (NRC) in 1993. The designer of the core of that reactor is on of Don Wolf’s teammates. Advanced Reactor Concepts (ARC) got their start in 2006. General Electric had a reactor called the PRISM, which was taken to the NRC in 1987 and five years later, and got a report with no impediments to licensing. They pursued the market for disposition plutonium, since arms agreements resulted in lots of fuel coming back. In 2008, Bill Gates formed Terrapower, a company developing a much larger reactor using the same technology, prototyped at 600 MW. A fourth U.S. company is pursuing this technology, but on a smaller scale. This represents an opportunity for the U.S. to take a lead in this technology.
0:00 – Economies and Diseconomies of Scale
Bret Kugelmass: Does the size of your reactor improve the economics of the technology?
Don Wolf: There are economies of scale and diseconomies of scale. The water-cooled reactors got very big and have very complicated safety systems. Today’s new reactors are safe, but they get safety by add-on engineered safety systems, making it more difficult to miniaturize. This technology doesn’t have the add-on systems, as it is inherently safe, and is a simpler design which could be done on a 100 MW scale for $350-500 million. There are advantages to being smaller, mostly related to the number of reactors that could afford it. Bigger reactors are more difficult to finance. Smaller reactors can be added subsequently to the market and there is not as much construction on interest, compared to large projects that last 5-8 years with long term construction interest. This will upset the diseconomies of scale. Advanced Reactor Concept’s reactor can compete with fossil fuels in a normal market to provide a clean energy source that provides a baseload source of power for renewables.
0:00 – Canadian Reactor Licensing Process
Bret Kugelmass: What level of technology development has your team progressed to so far?
Don Wolf: The technology right now is a largely finished conceptual design. The stages of design for a reactor starts on a bar napkin, then drawings are developed, models are created. Right now, the prototype can have modeled safety and heat. It is currently going through licensing in Canada. The U.S. licensing process is working to make the U.S. licensing process hospitable to new technologies, since most of the U.S. reactors are large water-cooled designs that the Nuclear Regulatory Commission (NRC) knows how to license and regulate. They have not had any experience with advanced reactors since the 1990’s. The Canadians offer a two-stage approach to licensing. The first is a vendor design review, which allows a firm with a conceptual design that doesn’t have a customer or operator to submit their design to the review of the Canadian Nuclear Safety Commission Staff, which is less expensive than going through the U.S. process. This allows a firm to get a letter after 2-3 years saying there is no impediment to licensing in Canada. Canada’s design review process is not technology specific; they take a risk-based approach. If they haven’t seen a feature before, they ask for the firm to prove it out, instead of requiring specific features that may not be needed in the new technology. There is risk reduction through an affordable capital investment and a better chance at having a time sensitive review. Canada licenses, the operator, site, and technology. After this reactor is built, the goal is that future versions of the technology are built throughout the world and licensing by performance, instead of by paper and analysis. Once it is working in Canada, regulators will be invited to take a look, taken through a repeat of the original safety demonstration, and then become licensed. This will open up the market for the technology’s value, being small in size, a 20 year fuel cycle, and very resistant to proliferation. The fuel doesn’t ever get out of the reactor into potentially hostile hands. An international group would install the reactor and 20 year fully loaded core. Any equipment used on-site to install and extract the rods would be taken off-site. After 20 years, the reactor would be shut down, and after a short cooling period, the team would return to extract the fuel rods, take them away, and install the new core. The sodium does not become extremely radioactive; the radioactivity that is present has a very short half-life. During decommissioning of EBR-2 after 30 years of operation, the sodium became inert after sitting for a few months and they were able to dispose of sodium easily.
0:00 – Global Interest of the U.S. in Nuclear
Bret Kugelmass: What are some of the challenges you anticipate going forward?
Don Wolf: The biggest and most important challenge is convincing the regulator that there is the data and theory to demonstrate inherent safety. The data comes from work down over time at EBR-2 and Idaho National Lab is working to bring the fuel data up-to-date. Once the data is available, it can be used during licensing in Canada in the next 2-3 years. Upwards of a billion dollars must be raised to get through the licensing process. Approximately $300-500 million will be enough to get a license working with a customer to break ground. The first of a kind build is going to cost $350-500 million funded by a rate of return from a power purchase agreement. This solution is a game changer to the problem of carbon. Electricity can be generated cleanly, but it is an approach that can be taken to other sources of carbon such as industrial processes and water desalination. There is a basic U.S. strategic interest to maintain high standards of safety and security of proliferation. Advanced nuclear is being worked on by the Russians and the Chinese. The U.S. needs to leapfrog over these two and get its technology into the market, for strategic and standard-setting reasons and to get the U.S. supply chain going again.
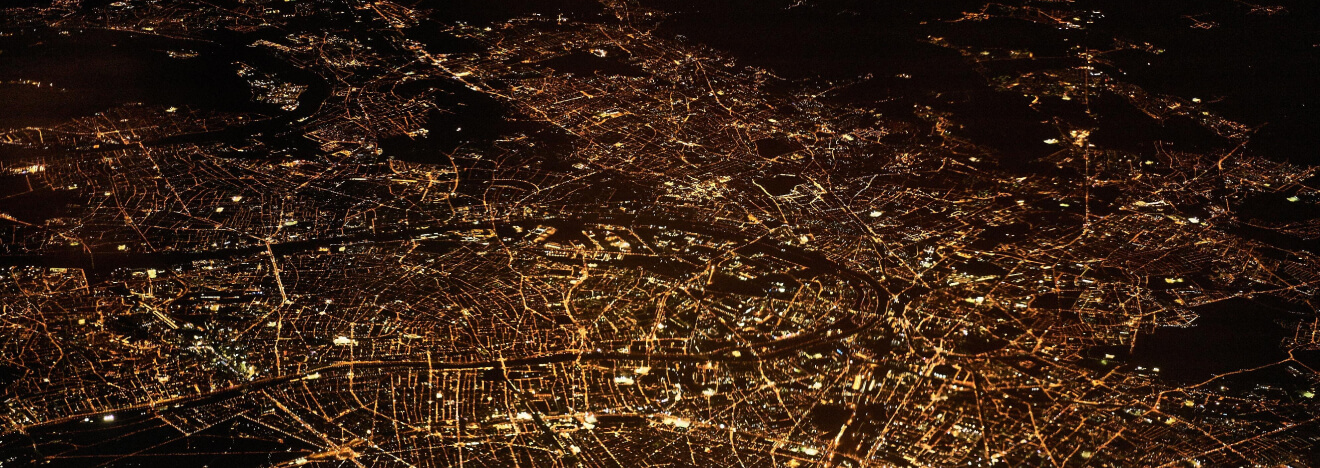