Ep 406: Dan Kotlyar - Associate Professor, Georgia Institute of Technology
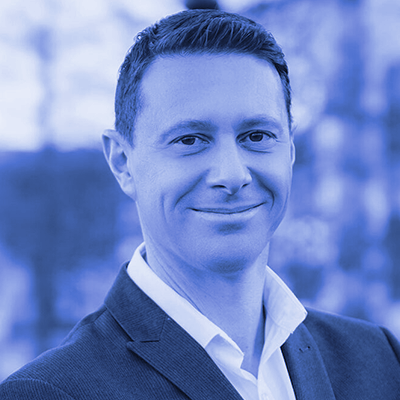
Show notes
Sarah Howorth [00:00:59] Welcome back to another episode of Titans of Nuclear. Today, I am in person at the Georgia Institute of Technology sitting down with Dr. Dan Kotlyar, and he is a newish-Associate Professor, I've heard. And he is working in the Computational Reactor Engineering Laboratory, also known as CORE. Dan, welcome.
Dan Kotlyar [00:01:22] Hi.
Sarah Howorth [00:01:24] It's so nice to have you on. I'm excited to chat with you today. So before we delve into your current work, let's just go ahead and talk a little bit about your background. Where are you from?
Dan Kotlyar [00:01:34] Oh, that's a tough question. So I'm from Israel, originally. Well, born in the Soviet Union, immigrated to Israel. I did my PhD there, and then had a journey to being postdoc at University of Cambridge, in England. And then, I was offered this position at Georgia Tech, and now I'm here.
Sarah Howorth [00:01:56] That's awesome. And so, did you do your schooling at all of those places, or is that where you worked in other professor jobs?
Dan Kotlyar [00:02:06] Well, I did my undergraduate, masters, and PhD in slightly different topics, but most of them were in nuclear engineering. My undergraduate was partially with electrical engineering as well. But I decided that nuclear was much more fun, I would say. Much more exciting. And so, that's where I've done my schooling. I actually became a part time lecturer, even during my PhD. I found that to be extremely interesting. So, I developed a passion for teaching. I've been doing this at Ben-Gurion University. That's the university in Beersheva in the south of Israel. I applied for multiple places, and one of the places where I really wanted to live was in Europe. Cambridge sounded like a great place to be at that time, and so I went and did my postdoc. No schooling, just research work. And I was mentoring students at Cambridge University, but also doing my research there.
Dan Kotlyar [00:03:16] Since I came here, what I do as an Assistant Professor, and recently, as an Associate Professor, is to teach students. Mainly, I teach reactor physics courses and reactor engineering courses. And recently, I started doing an experimental lab, which is even better. All that theoretical stuff is a bit boring sometimes. And I guess, my lab is doing the research with me on topics associated to reactor physics and reactor engineering.
Sarah Howorth [00:03:49] So, let's take it back a bit. What led you to the decision to pursue nuclear?
Dan Kotlyar [00:03:55] Oh, it's an interesting question. I'm not sure I have an easy answer.
Sarah Howorth [00:04:02] That's okay.
Dan Kotlyar [00:04:02] When I was 18 years old, you know, we go to the army first, and then we graduate with the army and then we go to university. I honestly didn't know what I wanted to do. I knew I wanted to become an engineer. I was sort of good in math and physics in my high school, and I decided to pursue a path, an engineering path. But what type of engineering, whether mechanical or electrical, I didn't really know. I think that's what pushed me towards this. It was not dual, but it was nuclear engineering with a major in electrical engineering, which is a bit odd, I think, right now as I'm saying this. Most of my courses were actually electrical engineering, and I started doing research in my junior year, and that's what I actually promote here at Georgia Tech, as well.
Dan Kotlyar [00:04:50] I try to encourage our undergraduate students to do research with any faculty in Georgia Tech. And so, I started doing my research in nuclear engineering. It was energy-related fuel cycle analysis of typical light-water reactors commonly used in our industry. You know, I fell in love in, I guess, with nuclear for energy, I would say. And so, I've sort of abandoned the electrical field, although some of the subject helped me later on as a professor, even.
Sarah Howorth [00:05:26] Awesome. And so, what are you specifically teaching here at Georgia Tech now?
Dan Kotlyar [00:05:30] Well, I teach reactor physics and reactor theory, which are, I guess, a continuation of courses, like the fundamental of reactor physics. Students are supposed to understand the basic mathematical models to link between the theory that sort of describes how a reactor would behave, what the reactor would look like, and why we design the systems in such a way. And then, we dive into more practical applications and the the mathematical level would increase in depth, but also their understanding of how the systems actually operate. Either this in sort of like a nominal operation or off-nominal, for instance, accident scenarios or something along those lines. It doesn't have to be an accident; it could be some type of off-nominal perturbation, I would say. And the students would gain that understanding during my classes.
Dan Kotlyar [00:06:32] Another course that I've taught for two years now is the graduate reactor engineering course. And this is sort of like a broad but in-depth overview of the specifics of light-water reactor systems. Most of our reactors are pressurized water reactors or boiling water reactors, so we typically focus on those. We do have advanced systems, as well, that we teach. But if you sort of understand how the light-water reactor operates, it gives you enough tools to understand other systems, as well.
Dan Kotlyar [00:07:03] And so, they dive into systems of how would you do fuel management, for instance, in the system? Why would you do this in such a way from an efficiency point of view? But they also understand things like how would the fluid flow, in this case, water flow, inside the light-water reactor? And they would try to understand the thermodynamic efficiency and why we design systems in such a way. And that is taught in the reactor engineering course.
Dan Kotlyar [00:07:33] And recently, I was asked to teach an experimental reactor physics lab, which I found to be even more entertaining for myself as a professor. Maybe not necessarily for the students as much; they have to do a lot of work there. But it's sort of going to the lab and trying to link the theoretical model that we teach during the reactor physics classes to actual applications in the lab itself.
Sarah Howorth [00:08:04] Let's narrow in and focus on that a little bit. Would you say that's your favorite area to teach?
Dan Kotlyar [00:08:11] The experimental reactor physics?
Sarah Howorth [00:08:12] Yeah.
Dan Kotlyar [00:08:12] I mean, recently it became my favorite area because my background is reactor physics, really, and eventually all my students would have to apply that knowledge during the research, I would say. Reactor physics, the core reactor physics, the hard core reactor physics, where they need to understand different computational methods because those methods would allow them to sort of design a better system, I would say, with less assumptions, maybe, and better safety margins, for instance. So, that's why reactor physics is still my favorite course.
Sarah Howorth [00:08:50] That's awesome. And I also hear that you're very interested in space. So, let's talk a little bit about nuclear thermal propulsion and rockets and all of that good stuff.
Dan Kotlyar [00:09:03] Yeah, we can. Is there any specific that you want to talk about? Because I can start now and probably, that would be our last discussion point here.
Sarah Howorth [00:09:13] Let's start delving into that a little bit, and just go ahead and start the beginning with what interests you the most. It's probably tough to zero in on that, because there's so much to talk about.
Dan Kotlyar [00:09:27] Let me just just comment on my background again and provide like a background story before I start with, specifically, the space propulsion system. So my background in general is either designing advanced systems, fission systems... I'm focused on fission systems, mainly. And those would be different Generation IV reactors, for instance, or even our own in-house developed systems that we are trying to develop here. They can either provide a benefit to an existing light-water reactor system, or even we can focus on a light-water reactor system and develop a better fuel cycle that may improve the fuel utilization, for instance. Meaning that the cost of your fuel would be cheaper, essentially. The cost of the fuel cycle would be essentially cheaper.
Dan Kotlyar [00:10:22] That is something that we do with companies that we work with. So for instance, my group works with Framatome to develop and push industry interests in developing a better fuel cycle. I guess up until four and a half years ago, I honestly never heard of nuclear thermal propulsion before. And so as I said, I encourage my students to pursue their research goals and get some research experience during their junior and senior year as undergraduate students. And if they like it, they can either pursue through their masters or PhDs, or they develop... The worst scenario, they simply have some research experience and they go to the industry or to the National Labs with some tools. I guess it's a win-win situation for everybody.
Dan Kotlyar [00:11:16] And I guess five years ago, a year and a half after I started at Georgia Tech, a group of undergraduate students after one of my reactor physics classes came to me and said, "Well, have you heard about the nuclear thermal propulsion concept? And would you be willing to mentor that project?" And I said, "Well, I know nothing about that. But yeah, most definitely. Let's do this." And so, they came up with all the literature review. They taught me most of the stuff that I know today, I guess. And of course, it evolved since then. But I've discovered a very interesting reactor concept. But even more than that, it's not really a reactor concept because, in reality, there were 20 systems, nuclear thermal propulsion systems that were built and tested, ground tested.
Dan Kotlyar [00:12:08] This is sort of known as... And this now brings me to that topic... Nuclear thermal propulsion had golden years from 1955 until 1973 under what is known to be the Rover Program. The Rover Program, I believe, was initiated at Los Alamos and it was sponsored by the US Air Force and the Nuclear Atomic Commission. And the main idea was to develop a program, or advanced nuclear thermal propulsion program, for ballistic missiles. But soon, they realized that they can use the same technology to send crewed missions to Mars. So, that's sort of like the background here.
Dan Kotlyar [00:13:03] And what happened after that is, I guess, the Rover Program had multiple sub programs. One of those was known as the NERVA program. So a lot of the times, Rover/NERVA... NERVA is, let's see, the Nuclear Engine for Rocket Vehicle Application. The objective of the Rover Program was to demonstrate the feasibility of a nuclear thermal propulsion engine. They had multiple sub-programs. As I said, they built 20 systems. They ground tested those systems as well. And I will just mention a couple of those, because those are sort of the milestones for today's programs that we have in the United States, mainly the United States.
Dan Kotlyar [00:14:09] So some of those programs, the first one was... They were all exotic fruits; I'm not entirely sure why. So, the first was Kiwi. The second one was Phoebus. And the third one that I will mention would be the Pewee. And again, as I said, most of today's systems sort of borrow some characteristics from those previous ones.
Dan Kotlyar [00:14:35] So the Kiwi 1, for instance, was the first series of reactors, nuclear thermal propulsion reactors. Again, nothing was tested in space; everything was ground tested. The Kiwi started with developing the first set of reactors. Kiwi-A, I believe, was tested in 1959. A while back, and it operated only for 5 minutes. It was operated at a power level of 70 megawatts. So we'll just give you a context, a typical light-water reactor, for instance, operates at anywhere between 3,000 and 4,000 megawatts thermal, and this system operated at 70 megawatts. This is a much smaller system and only for 5 minutes, although a light-water reactor, for instance, would operate for years under the same fuel. So here, we are looking at almost like a pulse type of operation, because we don't need to operate that system for a long period of time.
Dan Kotlyar [00:15:44] It operated for 5 minutes, and they did a post-analysis of the fuel and they discovered that the fuel was correct, that fuel reacted with the hot hydrogen. I'll explain why we used the hot hydrogen in a bit. And some of the fuel was lost. It moved through the fuel elements and was lost. Obviously, that's something that you wouldn't want to have. And so, as I said, there was this first series. The second series, Phoebus, was meant to improve some of those lessons. And so, they used coated particles, for instance. And again, those are very high temperature applications.
Dan Kotlyar [00:16:23] I'm probably jumping from subject to subject. I'll finish with this and I'll give you some context of why we need to have high temperature, as well. So Phoebus, for instance, operated for 10 minutes now. And it meant to operate at much higher power, because the idea was to demonstrate the feasibility of a nuclear thermal propulsion system. Instead of the 70 megawatts, it operated at 1,000 megawatts. A much higher power rate. And at that time, it set a record of having a propellant temperature reaching slightly less than 2,300 Kelvin. That's a very elevated temperature. And the fuel peak temperature, I believe, was slightly less than or around 2,400 Kelvin, which again, is enormous, in my opinion.
Dan Kotlyar [00:17:18] And this the third series which was an improvement to both was tested with the Pewee series of systems. And a lot of today's systems are actually based on that series. Pewee was the smaller scale of Phoebus. It was designed at 500 megawatts, and it set a record of 2,550 Kelvins in propellant temperature and 2,750 Kelvins in terms of the fuel peak temperature, which is again, extremely high. We don't typically operate reactors or our light-water reactors under such high operational conditions. So, why do we need to operate these systems? I guess I would have to explain a couple of terms here.
Dan Kotlyar [00:18:15] The metrics to define a propulsion system would be trust what we know, thrust and specific impulse. Thrust is we essentially have a certain propellant. We want to accelerate that propellant and then expel that propellant from a nozzle. That's what creates the thrust. And with nuclear thermal propulsion, you create the thrust by hitting a light propellant. In our case, that would be a hydrogen propellant, the lightest you can go. And it turns out that the lighter propellants have a much better efficiency in utilizing that propellant. So, thrust is one.
Dan Kotlyar [00:19:02] The second metric would be the specific impulse. But I'll first of all explain what is an impulse. Impulse is simply you take the thrust, and you sort of integrate this over time. So, it's the total thrust. The total thrust from the ignition of the engine until through its operation. That would be the impulse. The specific impulse is when you take this total thrust, the impulse, and you divide by the amount of propellant needed. And this metric provides you how efficiently you are utilizing your propellant.
Dan Kotlyar [00:19:35] And it turns out that the specific impulse behaves... It has two main contributors to how the specific impulse would behave. The molecular mass of the propellant itself, and the exit temperature or what we call the chamber temperature of the propellant when it exits the reactor. So the higher the exit temperature is, the better is the specific or the higher is the specific impulse, so the better is the utilization of the propellant. And the lower the molecular mass, again, a better propellant utilization.
Dan Kotlyar [00:20:16] So in principle, just to summarize, what you really want to do is you want to use light propellant, such as hydrogen, and to heat it to extremely high temperatures. Typically, we want to heat the propellant to a temperature of around 2,700 Kelvin.
Sarah Howorth [00:20:40] Wow.
Dan Kotlyar [00:20:41] Right. It's very high.
Sarah Howorth [00:20:42] Yes, yeah.
Dan Kotlyar [00:20:44] Which implies that most of your structural materials would sort of exhibit even a higher temperature, which poses a design challenge, I would say. Part of what my group does is develop computational methods to analyze such systems. Because nuclear thermal propulsion is a unique reactor, and for a unique system, you need a set of specific tools to be able to model those. And that's exactly what my lab is trying to do. We actually work with BWX Technology, and I can tell you more about that later on.
Dan Kotlyar [00:21:18] So this is again, the background of why, basically, we want to get the high temperature. And if I compare the nuclear thermal propulsion to other means of technologies that exist... So, you have the traditional chemical rockets that rely on combustion, but it turns out that nuclear thermal propulsion can provide comparable thrust levels. However, that specific impulse, that efficiency metric could be doubled, which means that you can have your traveling time to Mars.
Dan Kotlyar [00:21:56] That's why nuclear thermal propulsion is sort of looked at, at least now, as a promising technology. Because the distance from Earth to Mars is around... Well, it ranges between 50 and 400 million kilometers. It depends on the cyclic period, which changes every 26 months, I believe. But the idea is you have a large distance to cover, and so it poses a challenge, and a dose, a specific dose for the crew. So if you now want to send a crewed mission, ideally, you would want to minimize their exposure. And so nuclear thermal propulsion, as I said, can provide the same, thrust levels as chemical, but get you to Mars and back much quicker. That's just some background.
Sarah Howorth [00:22:59] Yeah, that's incredible. And you mentioned the design challenge of having those really high temperatures. Are there some current approaches to solving that challenge or is that still kind of an unknown area?
Dan Kotlyar [00:23:18] First of all, there are multiple players that are looking on advancing this technology. As I said, this technology was developed by the Rover Program. It was a slightly different fuel, but they did look on different fuel forms. So, they looked on ceramic fuels, and recently, the industry was looking into using ceramic metallic material, which allows you to reach those high temperatures. This is typically associated with a couple of, I guess, different considerations. One would be the properties of the materials. I don't know how deep I should go there, but you want to have...
Dan Kotlyar [00:24:09] First of all, a nuclear thermal propulsion system, it's really different from a light-water reactor. You need to satisfy different objectives. In the light-water reactor, you want to provide constant power for a year and a half between reloads, for instance, of the fuel. Ideally, you would want to increase the power because you can basically improve the fuel cycle costs, but not to make it too complicated to understand, the idea is that you work way below the... I'll try to rephrase myself here.
Dan Kotlyar [00:25:04] The idea is that you want to load the fuel, and the fuel provides you the energy. So, you don't want the fuel to be depleted too quickly because you would have to reload the fuel more frequently. And you want to operate for, let's say, a year and a half or maybe 24 months, for two years. Here, the operation is slightly different. You have different missions, but those missions typically have different maneuvers where the nuclear thermal propulsion would sort of satisfy those. In principle, you would have, for the outbound journey, two trans-Mars injection maneuvers, and then a Mars insertion maneuver where you would actually operate the engine. At all other times, you would have coasting of your vehicle, sort of speaking. You don't operate, so you don't have to turn on the vehicle or the engine.
Dan Kotlyar [00:25:58] And the last maneuver is the trans-Earth injection. So you have, basically, four maneuvers, and the system really operates for about 2 hours; that's it. So, you don't have that concern of sort of depleting the fuel. Because depleting the fuel is associated to how long you would be able to operate that.
Dan Kotlyar [00:26:19] Here, the objective is to satisfy that very high-temperature application. So it's completely different from a standard light-water reactor application where you want the fuel to be spent in the core for about a year and a half or two years. Here, you want that to operate for two years, and you want to squeeze as much power as you can to get to those very high temperatures. Make sense so far, right? So what was your question?
Sarah Howorth [00:26:50] It was just about how the design challenge of this really high heat is being approached.
Dan Kotlyar [00:26:58] Right, right. So here, what you want to do is you want to have a material that has a high loading of uranium, what we call the heavy metal loading. But you also want to use a material that has very good thermal properties. So with high conductivity, for instance, would be one of them. Because you almost don't want to create... Because as I said, the propellant temperature eventually would reach a temperature of 2,700 Kelvin, which means that the fuel would be hotter. If the conductivity of the fuel is very low, then you can breach and violate the safety of your fuel. You can melt it, for instance.
Dan Kotlyar [00:27:46] NASA is leading that or was leading that, and has multiple industry partners like BWX Technology who are looking on manufacturing the fuel and testing this fuel in different facilities in the United States. One of them would be the Transient Facility in Idaho National Lab. They've already manufactured the fuel. They're in, basically, two main forms of fuel that they are investigating. One is a ceramic material. And again, they're probably multiple variations. I will just tell you what I know at this point. Those are uranium nitride particles coated with zirconium carbide put in a zirconium carbide matrix. This is what I call the ceramic-ceramic. And you also have a refractory matrix, which is typically a tungsten matrix that holds those particles. And those are really the options that are being investigated right now. Uranium nitrate has this high uranium loading into the system. So, you load a load of that fissile material, essentially, and that's the first benefit. And second of all, the properties, the thermal properties are also enhanced when you compare them to a traditional uranium dioxide fuel, for instance.
Dan Kotlyar [00:29:10] I do want to say that during the Rover/NERVA program, they were using highly-enriched uranium fuel for their designs. And yes, we are borrowing a lot of the characteristics from the previous systems, but one of the main changes that was made was the choice of fuel. The choice of fuel now, with the discussion of proliferation, but also with the economic benefits of manufacturing multiple engines, eventually, led us to adopt the high-assay, low-enriched uranium. In less complicated words, enrichment of below 20% rather than enrichment that was previously used of above 90%. And this leads to a major modification to the previous series of reactors, like Pewee, for instance. Now we have to change the design itself to accommodate for the fact that we load less fissile material, if that makes sense. And we still need to operate for those two or maybe slightly more hours of operation.
Sarah Howorth [00:30:24] Awesome. And so looking into the future now, when do you see this technology coming to fruition and how will it impact the rest of the industry as a whole?
Dan Kotlyar [00:30:35] It's an excellent question. Obviously I don't build them myself, so I'm not entirely sure when. NASA was involved in this since when I came to Georgia Tech and I started doing this research with my undergraduate students, and after that, with a lot of my graduate students. I think that at Georgia Tech, I had more than 20 students involved in this project in the last six years, which is very impressive, in my opinion. Undergraduate students and graduate students. Apparently, a flying nuclear reactor makes students come to my lab.
Sarah Howorth [00:31:09] That's awesome.
Dan Kotlyar [00:31:09] Yeah. NASA had a big project to develop this technology and to revive that technology, I guess. And recently, there was an announcement made that NASA and DARPA joined forces to develop and deploy this technology, a prototype technology, by 2027. So coming back to my previous story, when those students came, I only looked at this as something that interested me from the research point of view. How would you analyze those systems? What would be the benefit of those systems? But now, I mean, it seems like there is a real chance that the United States will build its first flying nuclear system by 2027. And that system, from what I understand, would not really be used for Mars applications, but only be used as a flight demonstrated vehicle.
Sarah Howorth [00:32:18] Well, I think those visions for the future are a great note to end on, unless you have anything more to add that we haven't covered yet.
Dan Kotlyar [00:32:26] I think we've covered a lot.
Sarah Howorth [00:32:28] I agree, and that's awesome. Well, thank you so much for coming on. It was a pleasure to have you.
Dan Kotlyar [00:32:35] Thank you very much for hosting me.
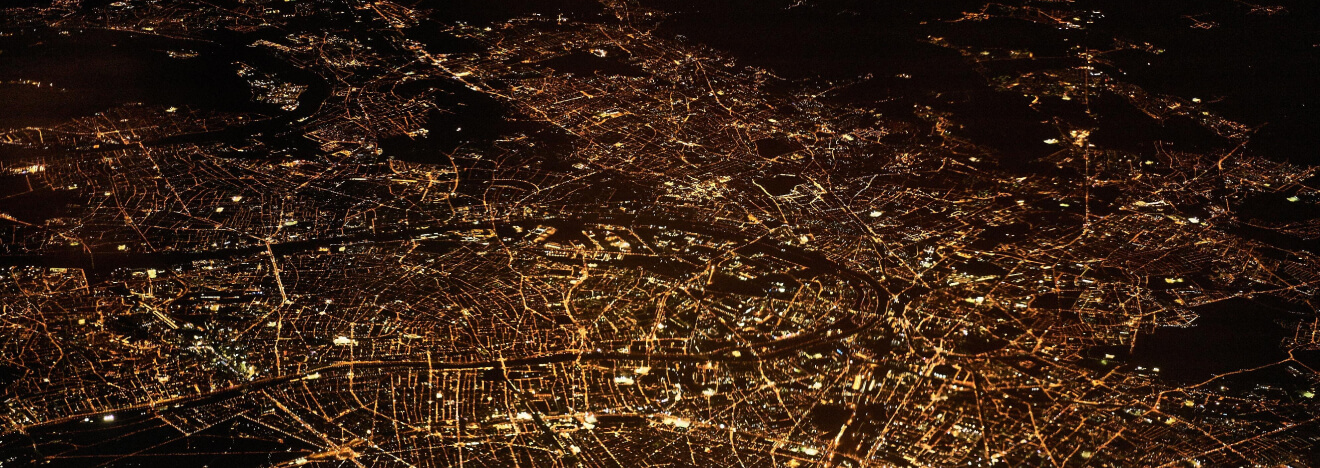